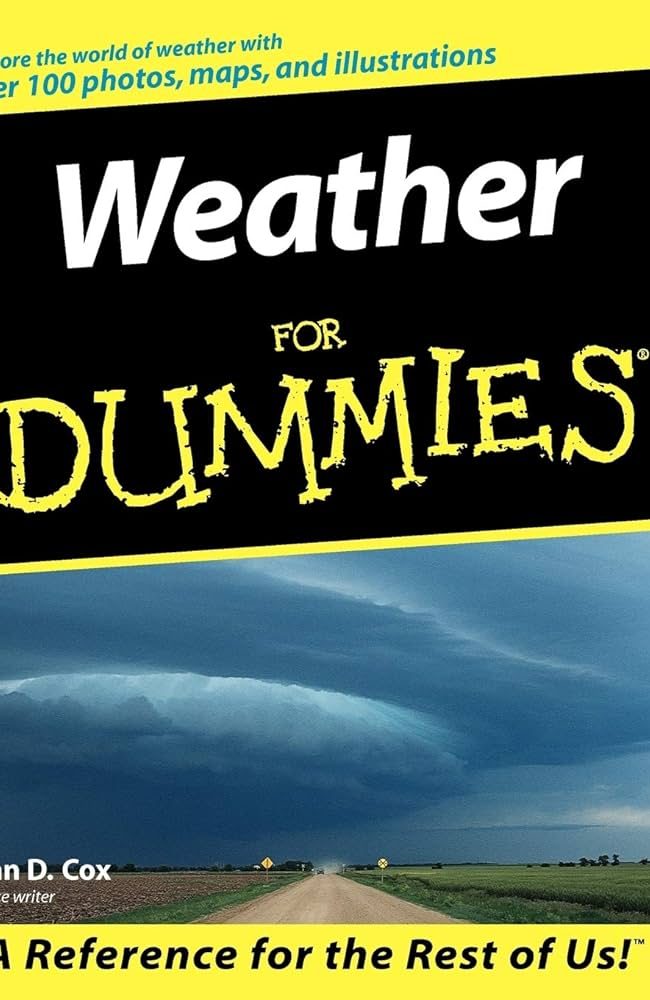
Weather For Dummies
John Cox
GENRE: Miscellaneous
PAGES: 472
COMPLETED: October 21, 2024
RATING: 




Short Summary
How do clouds and storms develop? Why is the sky blue? How are human activities contributing to global warming? In Weather For Dummies, John Cox answers all of these questions and more, helping readers understand one of life’s constants: the weather.
Key Takeaways
No Water Vapor, No Storms — The process for making a cloud and storms is as follows: water vapor, carried by warm air, rises, cools to its dew point, condenses into rain drops and ice crystals, and forms a cloud, which releases its contents. The more water vapor (i.e. moisture) in the air, the better the chances of clouds and storms. Without water vapor, clouds, rain, and snow cannot form. For example — a place like Arizona, where the air is very dry and is constantly being pushed down toward the surface by high pressure systems, generally experiences very little rain. A place like humid Florida, where the air generally contains a lot of water vapor and is often rising thanks to low pressure systems, experiences a lot of rainfall and storms.
Weather Starts at the Surface — Although it might seem like weather forms in the sky and works its way down to us, much of it actually starts at the surface. About 51% of the Sun’s energy (i.e. sunlight) passes through our atmosphere and is absorbed by the surface. On land, this energy is actually reflected back into the atmosphere as heat, warming the air. Over the oceans, which cover 70% of the planet, the energy is absorbed and warms the water. This warm water heats the air just above it, and the warm air then rises. As the warm air containing water vapor cools to its dew point, it condenses into clouds, raindrops, and ice crystals, forming storms that often blow inland off the Atlantic or Pacific. Some of these storms, like hurricanes, are huge. In many ways, weather works ground-up.
Opposites Create Chaos — Air masses are large bodies of air above us. Different air masses have different profiles, or personalities. Some are warm, some are cold. Some are humid, some are dry. When two air masses that are drastically different in temperature and moisture level collide, intense weather can ensue. The greater the difference between air masses, the crazier the weather. This is why summer and fall are fairly tame — warm air masses cover most of the country. As a result, there are few cold air masses around to cause trouble. Compare that to the winter in the Midwest. Cold, dry polar air masses from Canada and Alaska march south and meet up with warm, moist air masses that were already in place. When this happens, the warm air containing water vapor rises up, cools and condenses into raindrops, ice crystals, and clouds, and strong storms can develop. Because the Sun heats the planet unevenly (the Equator is hot while the North and South Pole are freezing), the atmosphere constantly shuffles air masses around in an effort to achieve balance.
Favorite Quote
“The Sun's energy is the fuel, and weather is the result of the huge temperature differences between the Equator and the poles.”
Introduction
- About the Book — Weather For Dummies is all about the weather! Weather affects all of us every day, but the factors driving the activity going on in the sky are a bit of a mystery to most. This book is designed to help the average person understand what causes certain weather outcomes like rain, snow, heat waves, tornadoes, hurricanes, and more.
- About the Author — John Cox is a professional science writer. He studied biological and physical sciences at Harvard University and MIT as a fellow of the Knight Science Journalism Fellowship Program.
Ch. 1: Forecasts and Forecasting
- Meteorologists — Meteorologists study all of the turbulent and swirling gases in our atmosphere and try to make predictions as to how all of this activity will affect the weather we experience. In this quest, they are aided by the help of giant supercomputers that crunch complex numbers and complete weather models and forecasts. In addition to the information they receive from these supercomputers, local meteorologists can enhance the weather report by including their knowledge of things like nearby mountain ranges and lakes that can affect weather in the area.
- The National Weather Service — This is where local and national meteorologists get a lot of their data. The National Weather Service is located near Washington D.C. and hosts a small army of supercomputers that do the technical work that produces all of the weather forecasts we read and see and hear every day. Most of us do not see the incredibly complicated models and statistics that these supercomputers churn out, but these outputs are the most important ingredients in the making of the average weather report.
- Quote (P. 12): “Television meteorologists compete with one another for your viewing pleasure, with their different styles and presentations and engaging personalities, but they all have the computing and forecasting resources of the National Weather Service on their sides.”
- Quote (P. 12): “Day in and day out, the statistics that come out of large and powerful software programs run on supercomputers are the single most important ingredients in the making of the average public weather forecast.”
- Severe Weather “Watches” and “Warnings” — Anybody who has lived in a place like Oklahoma or Iowa is familiar with tornado watches and warnings, which alert the public to severe weather in the area. Both are issued by the National Weather Service. A watch signals that conditions are favorable for tornadoes or other severe weather outcomes like big thunderstorms, floods, blizzards, heavy snow, high winds, heat, and dust storms. A watch means something potentially dangerous could develop soon. A warning is issued when a tornado has been spotted or detected by radar, indicating immediate danger. In Iowa, this is when tornado sirens go off, and people head to their basements.
- Quote (P. 13): “The National Weather Service Storm Prediction Center issues ‘watches’ that alert the public to the possibility of trouble. But then it’s up to the local forecaster to keep a close eye on things. On the lookout for damaging hail, local flooding or tornadoes, she relies heavily on local radar observations and uses her own training, experience, and understanding of local conditions to decide whether to issue a public warning.”
- The Making of a Weather Forecast — There are several steps to making a weather forecast. Everything starts with feeding the computers located at the National Weather Service with accurate information about current weather conditions. From there, simulations take place that produce the forecasts we see on a daily basis. More details on the weather forecast process:
- Start With Now — The first step is to understand the initial weather conditions and feed these details into the computers at the National Weather Service. These details serve as the starting point for the computers. What is the atmosphere doing right now? How hot or cold is it? Which way is the wind blowing? Is it cloudy or clear? This information must be as accurate as possible, because even the slightest error in the description of current weather conditions can lead to huge errors in the overall forecast that the computer generates. To get the most accurate information possible, instruments such as weather balloons and airplanes, satellites in space, ships at sea, and satellites are used. Data from land-based weather stations around the world are also used.
- Quote (P. 14): “Everyday, 24 hours a day, data from thousands and thousands of weather observations around the world is streaming electronically into National Weather Service computers at the World Weather Building in Camp Springs, Maryland. With these millions and millions of bits of data, the computers are constantly updating and refining their highly detailed descriptions of the current state of the weather.”
- Predict the Future — In the second step, computer models divide the atmosphere over the surface of the Earth and above it into imaginary individual blocks, or gridpoints. Each gridpoint has values for key weather features like temperature, pressure, wind, and humidity. The models apply complex mathematical equations to simulate changes in these features over time. But each complete computation moves the atmosphere forward into the future only a few minutes. So the process is repeated over and over again. The experts say that to produce a weather forecast of a few days, modern computer models may need to complete more than one trillion calculations. Therefore, the forecasts we see every day are the results of a huge, huge number of computer simulations.
- Start With Now — The first step is to understand the initial weather conditions and feed these details into the computers at the National Weather Service. These details serve as the starting point for the computers. What is the atmosphere doing right now? How hot or cold is it? Which way is the wind blowing? Is it cloudy or clear? This information must be as accurate as possible, because even the slightest error in the description of current weather conditions can lead to huge errors in the overall forecast that the computer generates. To get the most accurate information possible, instruments such as weather balloons and airplanes, satellites in space, ships at sea, and satellites are used. Data from land-based weather stations around the world are also used.
- Interesting Fact — The word meteorology is a funny one for weather. Meteors are objects in space and don’t have anything to do with the weather. So why do we call the study of weather meteorology? Aristotle was the one who first used the word meteorology back around 350 B.C. In those days, everything that happened above the earth was known as astronomy, and Aristotle was trying to define a new science. He figured astronomy was the study of all the stuff that happens in the distant heavens, and meteorology was the study of the stuff that happens closer to Earth. We haven’t given it a new name since.
- Interesting Fact — The No. 1 weather-related killer in the United States is heat. The National Weather Service issues watches and warnings for severe heat. You see these on your weather app in Arizona.
- Aviation Forecasts — In addition to daily forecasts, the National Weather Service also prepares specialty forecasts. The aviation forecast is one of them. Communication satellites are used by the National Weather Service to deliver the World Area Forecast System, which is designed for use by pilots of commercial airliners. This tool gives them the best possible information about things like upper-level winds and temperatures. This data is crucial for pilots as it gives them key information on turbulence, icing, and thunderstorms. Also, many of the land-based weather stations around the world that contribute to daily forecasts are set up near airports. The readings from their instruments are automatically broadcast to pilots in real-time. The goal is to give pilots the best possible information to work with.
- Probability of Precipitation — Technically, the probability of precipitation (PoP) represents the chance, expressed as a percentage, that a measurable amount of rain — 0.01 inches or more — will fall somewhere in the forecast area during the forecast period. This percentage is a combination of two factors: the forecaster’s confidence that precipitation will occur and the portion of the area expected to receive rain. For example, a 70% chance of rain doesn’t mean that 70% of the area will get rain or that rain will cover the entire area. It simply indicates that there is a 70% likelihood that rain will occur somewhere within the forecast area. So, even though the forecast calls for a 70% chance of rain, you may not get any rain depending on where you are in the area.
- How Humidity Works: Relative Humidity — Humidity is the amount of water vapor in the air, and it plays a significant role in both how we perceive temperature and how the air behaves. In the western U.S., people generally experience a “dry heat,” while the eastern U.S. tends to have higher humidity levels. Relative humidity is expressed as a percentage, showing how much water vapor is in the air compared to the maximum amount the air can hold at a given temperature. For example, if the relative humidity is 40%, the air is holding 40% of the maximum amount of water vapor it could hold before reaching its saturation point. When the air reaches 100% humidity, it’s fully saturated, meaning it can’t hold any more moisture and will begin to release some of it in the form of condensation or rain.
- How Humidity Works: Dew Point — Some say that the dew point is a better measure of humidity than relative humidity. The dew point is the temperature at which the air becomes completely 100% full of water vapor (moisture) and can’t hold any more. When the air cools down to the dew point, the water vapor turns into liquid, forming things like dew on the grass or fog. A way to think of it: At <insert given dew point temperature from weather app> the air has reached 100% humidity and is 100% full of water vapor. Relative humidity is a measure of how much water vapor is already in the air. When the dew point is close to the current air temperature, the air feels more humid. This is because the air is almost completely full of water, making it feel sticky and harder for sweat to evaporate, so you feel hotter. If the dew point is far below the air temperature, the air feels drier, which makes it feel more comfortable. So, the dew point helps us understand how humid the air feels. A higher dew point means more moisture in the air, and it feels more humid, while a lower dew point means drier air.
- Quote (P. 65): “The key is the distance between the air’s temperature and its dewpoint temperature. If the distance is big, the air is dry, and chances are it won’t come near saturation and condensation will not occur. If the distance is small, the moisture content of the air is high, and you could be in for an uncomfortable day and night.”
- Why Humidity Feels Worse — Speaking practically, humidity makes a big difference in how we feel temperature. On a dry day, 95 degrees can feel tolerable because sweat evaporates easily, cooling your body. There is simply not a lot of water vapor in the air to block your sweat from evaporating and taking your heat with it. But on a humid day, that same 95 degrees feels much hotter because the high amount of water vapor in the air prevents sweat from evaporating, making it harder to get rid of heat and cool your body. A good way to understand this is to think about how the air feels after a hot shower. The high humidity in the bathroom makes the air feel much warmer because it’s saturated with moisture, much like what happens on a hot, humid day. When you leave the room, you feel a nice cool sensation; that’s the heat from your body being able to release without any issues. The quotes below help to understand humidity.
- Quote (P. 24): “Water vapor is an invisible gas, but you see and feel the effects of the air’s relative humidity all of the time. When you take a shower, for example, you’re adding so much water vapor to the air that it is quickly saturated. Condensation is producing tiny water droplets and you’re standing in a little cloud. Step out of the shower, and you might begin to feel a little chill. You are stepping into air that is less humid, and heat is leaving your wet skin through evaporation. The heat is used to convert the liquid into the water vapor that is being absorbed into the air. The bathroom mirror is fogged up because the air up against its cooler surface has fallen to its saturation point and some of its water vapor has condensed into dew. Clouds form when air rises and cools to its point of saturation.”
- Quote (P. 24): “Relative humidity on a warm day can make all the difference in how you feel. When the relative humidity of the 95-degree air is low, it is relatively easy for the body to cool itself. . . When the relative humidity of the 95-degree air is high, however, it [the air] really doesn’t want to take up much more water vapor, thank you, so it is much harder for the body to get rid of its heat and its perspiration. So the heat builds up, the perspiration accumulates, and the poor body, well, it just kind of stews.”
- The Heat Index & Wind Chill — The National Weather Service has a Heat Index that shows how hot you will feel given the air temperature and level of humidity in the area. The index combines both properties to deliver a temperature that your body will actually feel. Similarly, the National Weather Service has an index that shows how your body will feel during cold temperatures when there is wind. Wind makes the body feel cooler, both when the temperature is hot and when it’s cold. In the winter, wind chill is no fun — it makes temperatures that are already very cold feel even colder.
- Tools of the Trade — Although creating a forecast is complicated, there are really only three key properties of the atmosphere that are responsible for almost all of the weather that happens. These are: air temperature, moisture (humidity), and motion (air pressure). We use a variety of instruments in order to measure the current conditions in all three of these areas, and the data from these tools is sent to supercomputers that perform calculations, create models, and spit out a weather forecast. The most common instruments we use to measure current conditions are thermometers, hydrometers, barometers, and more. The problem is that we need to get these instruments into some tricky places in the atmosphere. This is where “instrument carriers” come in. Below are a few instrument carriers that help us get accurate data for the computers.
- Weather Balloons — Weather balloons filled with hydrogen gas are released from 1,000 sites, twice per day at 7 a.m. and 7 p.m. ET. Balloons are tracked by radar to measure wind speed and direction in the upper atmosphere. The balloons eventually pop at 20 miles up, and the measuring instrument falls back down into the ocean or on land.
- Ocean Buoys — Ocean buoys deliver readings on the temperature of ocean water, air temperature at sea, humidity, and air pressure.
- Automated Weather Stations — Across the U.S., there are about 1,000 land stations measuring surface weather conditions every minute. These stations are operating automatically 24 hours a day. These stations track cloud cover, visibility, temperature, air pressure, wind speed, and precipitation.
- Doppler Radar — The Doppler Radar is a powerful remote-sensing tool that detects thunderstorms that other instruments have a hard time tracking.
- Satellites — Prior to 1960, we didn’t have any satellites in space helping us predict weather. As a result, we were often very bad at detecting storms and didn’t report inbound dangerous conditions until it was too late. Today, we have many satellites up there taking photos of the weather from above. These images are taken and sent back to Earth in real-time, helping us detect severe storms.
- Computers — Again, computers are a huge part of weather forecasting. All of the data that our instruments collect is sent to these supercomputers. They take the data, run their calculations, and spit out forecasts, charts, and more. Over the last few decades, the National Weather Service has spent billions of dollars to improve the equipment we use to forecast weather, and a lot of that money has gone toward these computers.
- Chapter Takeaway — Producing a weather forecast is a complicated process. In a nutshell, in involves using a variety of instruments to gather accurate data, which is then fed to supercomputers located at the National Weather Service headquarters that crunch the numbers, run complex calculations, and produce a forecast. It’s crucial to get accurate information on current conditions — any misinformation can throw the forecast off significantly.
Ch. 2: Behind the Air Wars
- Living in the Middle: Fronts — Our atmosphere is very hot on one side and very cold on the other. It’s like living in a house where one end of the house is always hot (the Tropics at the Equator) and the other is always cold (the North and South Pole). On any given day, it can reach 100 degrees in the desert and 100 degrees below zero in Antarctica. Fortunately, most humans live somewhere in the middle. On average, it’s a lot more comfortable in these middle latitudes, but occasionally we get a situation where northbound tropical warm air meets southbound cold polar air. When this happens, it’s not a pretty picture. Big storms can break out — think of these storms as battles between armies of hot air and cold air. The lines between them are appropriately called fronts — as in battlegrounds. The following few bullets have more on fronts.
- Air Masses: Little Divas — Building on the point above, an air mass is a large body of air that covers hundreds or even thousands of miles. This air has almost the same temperature and moisture levels. Air masses have their own “personalities,” and when different air masses meet, fronts are formed and rough weather events like storms can occur. For instance, when the wind blows cold continental polar air masses south from Canada and Alaska, these polar air masses usually bring freezing temperatures to the Midwest and Northeast regions of the U.S. during the winter months. The Rocky Mountains in Colorado help block much of this polar air from reaching the Southwest and West Coast, which is why these regions tend to have milder winters. But in the summer, continental polar air from Canada and Alaska brings a cooling effect, which is why much of the Midwest and East Coast experiences nice summer weather while the West Coast gets hot. Other types of air masses include:
- Maritime Polar Air Masses — Maritime air masses hang out over the ocean. In the winter, maritime polar air masses sweep over the West Coast from out of the northern Pacific Ocean, bringing cool, moist air that dumps heavy snow in the mountains in the West Coast.
- Maritime Tropical Air Masses — These air masses hang out over the south Pacific Ocean and sweep northeast over California in the winter, bringing a lot of rain and flooding. Maritime tropical air masses from the Atlantic Ocean and the Caribbean Sea often get blown in the eastern part of the U.S. during the summer, bringing a lot of warm temperatures and high humidity.
- Continental Tropical Air — These air masses form over desert regions of north Mexico and the U.S. Southwest (i.e. Arizona) in the summer, keeping things hot and dry. Thanks to the shielding of the Rocky Mountains, Arizona doesn’t get a lot of continental polar air masses blown into it. And the state is located in a spot where maritime polar air masses from the Pacific don’t quite reach it. All of this contributes to the state having such a warm climate.
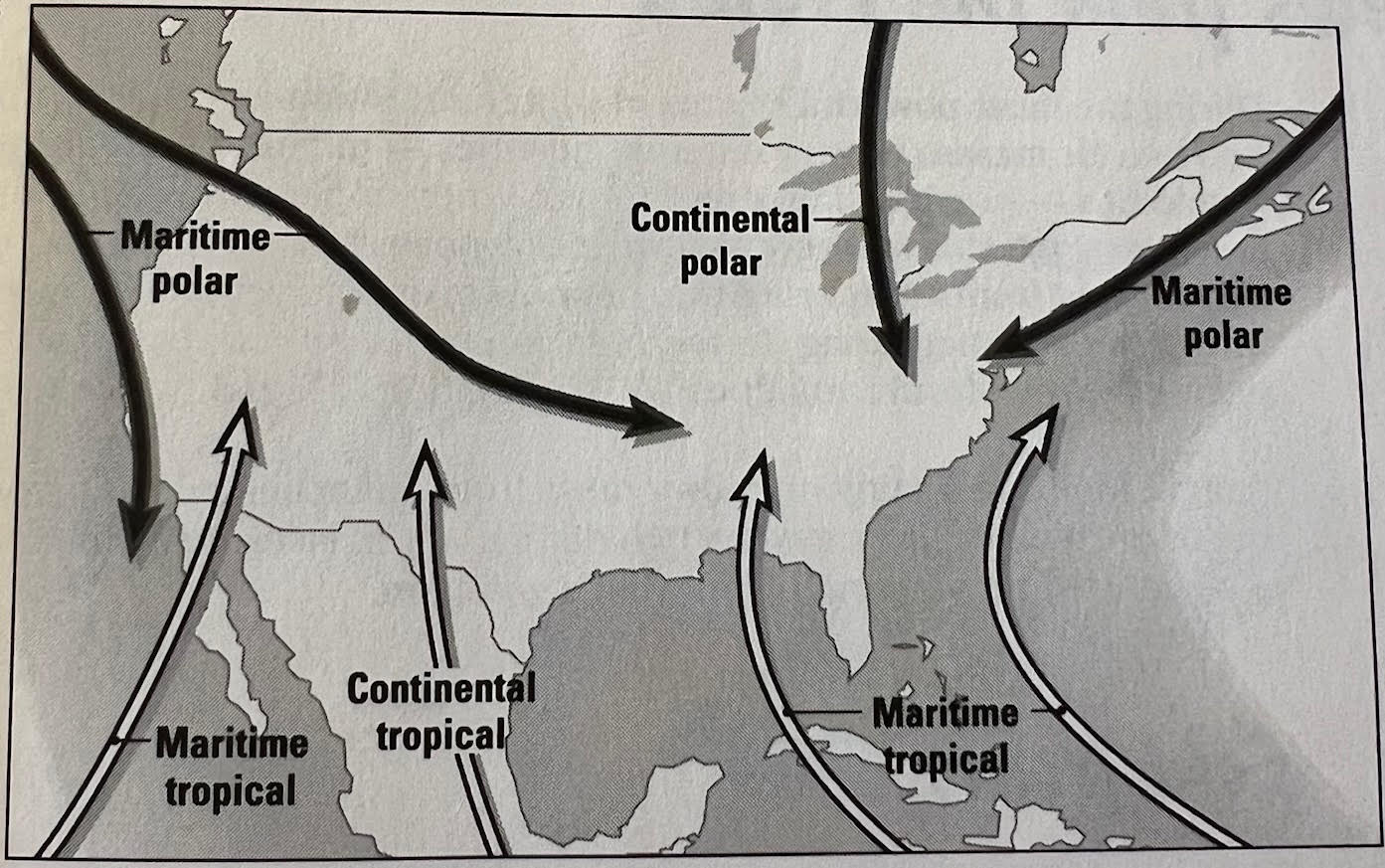
- Types of Fronts — There are two primary kinds of weather fronts: warm fronts and cold fronts. The “front” is just the name of the boundary or “head” of a cold or warm air mass. For example, a big warm air mass has a front boundary that separates it from other kinds of air masses. This boundary line is called the front. When a “cold front is coming,” this just means that a cold air mass is coming our way. The craziness of the ensuing weather will depend on how different the incoming cold air mass is from the air mass that is already overhead. When two very opposite air masses meet (i.e. a very cold air mass moves into a very warm air mass), weather battles take place between the two opposing fronts. Big storms are common in these situations. More on cold and warm fronts below:
- Cold Front — In a cold front, a cold air mass moves in and pushes beneath a warmer air mass because it is more dense (i.e. heavier), forcing the warmer air to rise quickly. As the warm air rises, it cools to its dew point and condenses into rain drops and ice crystals that form into clouds. These clouds can lead to intense weather conditions like thunderstorms, heavy rain, or even snow.
- Warm Front — In contrast, a warm front occurs when a warm air mass moves into a cool air mass. The warm air, being less dense (i.e. lighter), gradually slides over the cooler air, rising more slowly. This typically leads to a more gradual increase in cloud cover and light to moderate precipitation. Warm fronts can bring overcast skies and steady rain or drizzle that may last for longer periods compared to the more intense, short-lived storms of a cold front.
- Air Masses Collide = Craziness — When air masses of different “personalities” (e.g. temperatures and humidities) collide, battles between the two fronts develop and bring down severe weather. The blizzards and tornadoes and other random weather events that happen are a result of these opposite air masses colliding. For example, when a cold front (i.e. a cold air mass) moves into a region occupied by a warm front (i.e. a warm air mass), it causes a collision (i.e. a “battle”) between these very different air masses. This collision sends the warm air above the cold air. When this happens, the warm air (which always contains more water vapor than cold air) rises and cools to its dew point. When this happens, the air condensates into rain drops and ice crystals stored in clouds. The rain and snow inside these clouds is then released, resulting in stormy weather.
- Quote (P. 42): “During the most powerful storms of winter and summer you will probably find two air masses of very different qualities — different temperatures and humidities — crashing into one another. When upper winds and other conditions are right, this is where you will see a powerful mid-latitude cyclone with well-defined fronts that bring rain or snow over big sections of the country. In spring and summer, along these fronts can be long lines of severe thunderstorms that can form tornadoes when conditions are right — or wrong!”
- Quote (P. 72): “And sharply contrasting temperatures help make a lot of weather.”
- The Sun Drives the Weather — The Sun is the driver of the weather. Without the Sun’s warmth, the Earth would freeze over. The Sun’s energy gives our planet life, and weather is the result of the huge temperature differences between the Equator (hot) and the north and south poles (cold). Essentially, the atmosphere is always trying to move the Sun’s heat from a warmer region to a cooler region in an attempt to achieve balance. This process of trying to move heat around and achieve balance via wind results in air masses of different temperatures and humidities colliding, creating weather conditions like rain, snow, storms, tornadoes, hurricanes, etc.
- Quote (P. 44): “Weather is the Sun’s doing. It might be hard to believe when you are being hammered by a cold winter storm, but the Sun, the star of the solar system, is the driving force behind all this weather, the commander in chief of all these air wars. The heat energy — the solar energy — radiating from this star is the fuel that drives it all, everything I describe in Weather For Dummies.”
- Quote (P. 44): “The Sun’s energy is the fuel, and weather is the result of the huge temperature differences between the Equator and the poles.”
- Quote (P. 44): “The atmosphere is always in the process of converting the Sun’s energy from one form to another and moving it from place to place. Behind all of the motion, and commotion, is a complicated exchange of energy between the atmosphere and the Earth. Things are out of balance, and the system is trying to even them out.”
- The Sun’s Energy: Moving Ground Up — The Sun’s energy comes down to Earth as visible short-wave energy (i.e. sunlight), with about 50% being absorbed by the surface and around 20% being absorbed by the atmosphere and clouds. The energy that reaches land warms the ground and the air above it, is converted into invisible long-wave radiation, and is then rebounded back up toward the atmosphere. The ocean is different; it absorbs the Sun’s heat a lot better and doesn’t bounce sunlight back up. Most of the heat reflected off of land is then spread throughout the atmosphere via a process called convection, which involves warm air rising and cool air sinking. This convection process, combined with wind, moves heat to cooler regions and helps create weather patterns. Therefore, weather is influenced by both the heat being rebounded from the surface and the movement of air in the atmosphere.
- Quote (P. 45): “Through heat-transfer processes that weather scientists call conduction and convection, the radiation surplus is continually moving from the surface up into the atmosphere. The heat is conducted directly from the surface to the thin layer of atmosphere above it. You might say that the convection process takes it from there, mixing it through winds and other weather processes among the higher layers of atmosphere.”
- Quote (P. 45): “While weather watchers like you and I focus on the sky, on the rays of the Sun beating down on us and on the rain or snow falling from the clouds, a weather scientist sees things as part of an energy transfer that is moving from the ground up. A winter storm is transferring energy from the surface to the atmosphere . . . Through conduction and convection, they [weather events] are all moving one form of heat or another from a warmer region to a cooler one.”
- Quote (P. 48): “This invisible long-wave radiation that is given off by the Sun-warmed Earth is more important to weather than direct sunlight. Somebody who has spent the day in the sunshine may find it hard to believe, but a weather scientist will tell you: The energy radiating back up into the atmosphere from the surface of the Earth as long-wave radiation has more direct effect on weather processes than the short-wave energy that comes directly through the atmosphere as sunshine.”
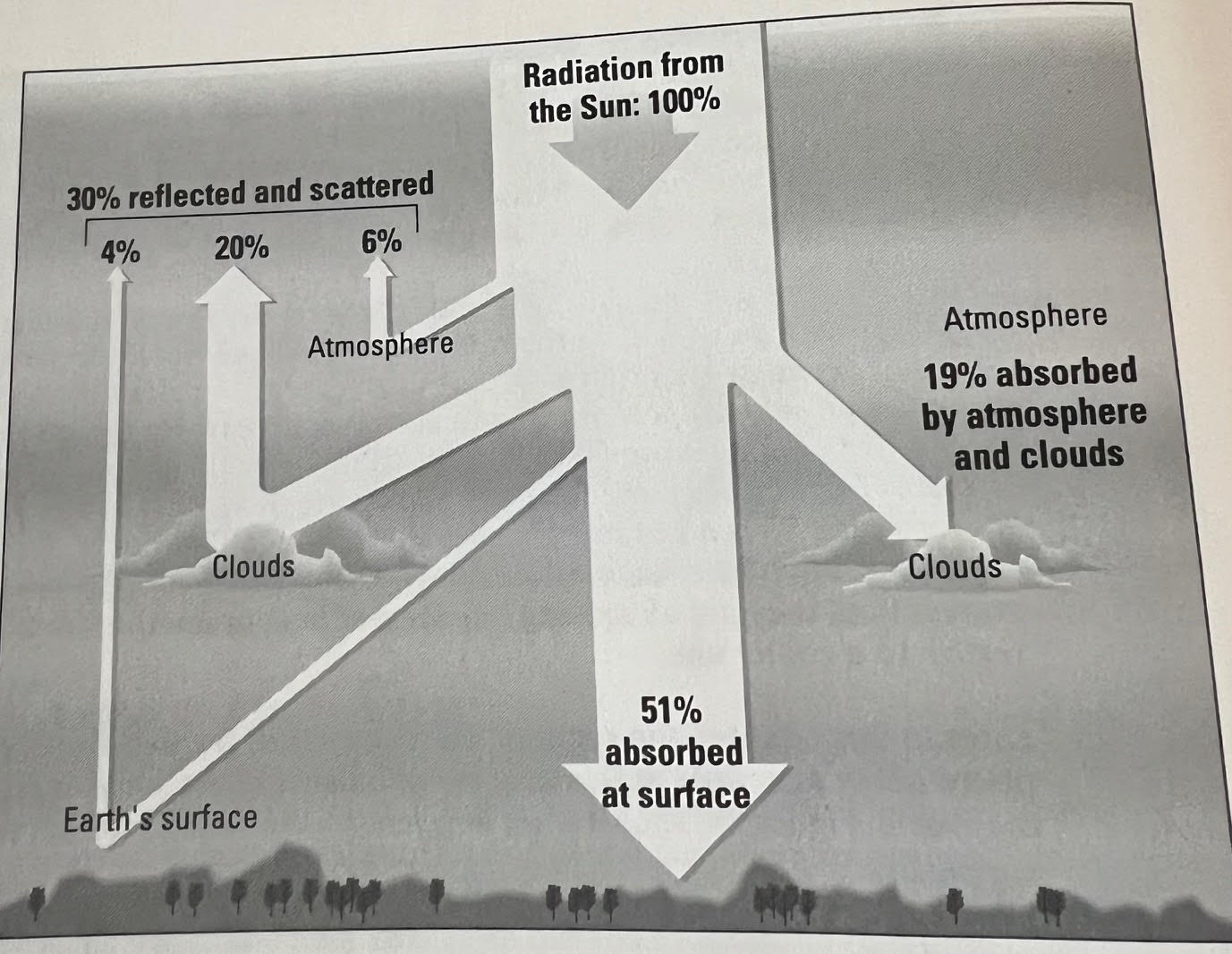
- Clouds Create a Warm Blanket — In addition to the Sun’s energy being rebounded back up to the atmosphere as invisible-long wave infrared radiation, everything on this planet with a temperature above absolute zero (including this cell phone!) gives off some form of radiation. As we learned in the previous bullet, this radiation has a lot to do with weather. Clouds especially like invisible long-wave infrared radiation. In fact, cloudy nights are often warmer than clear nights. This is because clouds are absorbing the radiation being sent back up from the surface and are sending it right back down to the ground, keeping us warmer like a blanket. This keeps the surface warmer than it would be on a clear night, where heat escapes into space easily.
- Interesting Fact — The brighter the surface of something, the more of the Sun’s light it reflects (and the less it absorbs). That’s why a white shirt is cooler than a dark one on a summer day.
- Reason For the Seasons — Earth is closest to the Sun on January 3 and furthest away from the Sun on July 3. On January 3, we’re receiving 7% more heat energy from the Sun because the Sun’s rays are traveling less of a distance and are more concentrated compared to July 3, when the Sun’s rays are more spread out because they are traveling further. So why is July so much hotter than January? It has to do with the tilt of Earth: as a planet, we are not standing up straight; we are tilted at a 23.5-degree angle. This tilt means that, in the winter, the Sun’s rays are striking us at a low, glancing angle, whereas during the summer months the Sun’s rays are coming down directly on top of us. It’s almost like the rays are hitting us from the side in the winter but are coming straight down in the summer. Without this tilt, we wouldn’t have seasons at all; everywhere on Earth all year long would have exactly 12 hours of sunlight and darkness every day. The tilt is why some parts of the year have more sunlight than others.
- Quote (P. 50): “Scientists have figured out that Earth gets 7 percent more heat energy from the Sun on January 3 than it does on July 3. This is because on July 3, even though it is mid-summer in the Northern Hemisphere, the sun’s rays are traveling a little farther and so are slightly more spread out than they are on January 3. But this small difference does not account for the seasons. The angle that they strike a particular place on Earth makes a lot more difference to the intensity of the Sun’s rays.”
- Quote (P. 51): “For most of the world, the winter Sun that comes glancing in at a low angle is a pretty weak sister to the summer Sun that spends a lot of time directly overhead.”
- Quote (P. 52): “This 23.5-degree angle is why you have seasons. This is the whole reason why there is winter and spring and summer and fall. This tilt is why there is a time of year when plants are growing vigorously and another time when they are dormant. This slant of the Earth is the reason why January 3 and July 3 have a completely different feel. And this is why some times of year the Sun races across the sky and sets like a falling rock and at other times it just seems to hang up there all day long.”
- The Atmosphere: Layers — The atmosphere protects us from the Sun’s ultraviolet rays; without it, we’d all be cooled. It starts at your feet and extends up about 80 miles. Over that 80-mile stretch, there are four main layers, starting from lowest to highest: troposphere, stratosphere, mesosphere, and thermosphere. The troposphere, 0-10 miles up, is where basically all of Earth’s sky and weather stuff takes place. The air just gets thinner as you go up because air molecules become very distant and spaced out from each other. This is why we need air-pressured cabins in airplanes; without them, there wouldn’t be enough available oxygen to be able to breathe. Also, about 78% of the air we breathe is nitrogen, only 20% is oxygen.
- Chapter Takeaway — In many ways, weather is driven by a ground-up process. The Sun’s energy travels down and through the atmosphere, with about 51% of it absorbed by Earth’s surface. This energy is then converted into long-wave radiation and rebounded back into the atmosphere. Through convection, warm air rises and moves toward cooler regions, while cool air sinks. This process is the atmosphere’s attempt to achieve balance. This movement of air can create fronts — boundaries where warm and cool air masses meet, which often lead to storms and other weather events.
Ch. 3: Land, Sea, and Precipitation — Is This Any Way to Run a Planet?
- The Water Cycle — About 75% of Earth’s surface is covered by oceans, and the oceans carry 97% of the planet’s total water. When it comes to making weather, the water is where a lot of the action is. During the water cycle, water changes form from gas to liquid to solid as it gets moved from the oceans, the land, and the atmosphere. Weather and the water cycle drive each other; they’re connected. There are many different routes water can take, but in a nutshell water from the oceans evaporates/rises up into the atmosphere, where it cools/condenses and is delivered to land through rain and snowfall. The cold of the winter stores some of it in the mountains as snow and ice. In the spring, it melts and comes down into rivers and lakes. Over the ensuing days, months, and years, it flows back to the ocean. Below is a closer look at this process:
- Ocean to Atmosphere — Water rises from the ocean and becomes part of the atmosphere by being transformed from its liquid state to its gas form, known as water vapor. This happens through the process of evaporation.
- Quote (P. 74): “Without the oceans, the weather would be just a lot of hot air. Well, that’s an exaggeration, but you get the idea. Land surfaces make good storms, but most of the rain and snow that falls is water vapor that has evaporated from the ocean . . . Up and down the U.S. West Coast, virtually all of the storms of winter are dumping Pacific Ocean water vapor . . . Warm ocean currents such as the Gulf Stream carry warm air that gives the U.S. southeastern coast a much more tropical feel than it otherwise would have.”
- Atmosphere to Surface — A molecule of water vapor evaporated into the air will spend about 7-10 days in the atmosphere before it cools to its dew point, condenses into a cloud, and falls back to the surface as rain or snow. Where the water lands plays a big role in how long it takes to get back to the ocean. If it lands on a hot parking lot, it will evaporate immediately and return to the atmosphere. If it lands as snow on a mountain, it may take hundreds of years for it to work its way back to the ocean.
- Surface to Ocean — For most of the water vapor in the atmosphere, the journey back to the ocean is very direct. Most precipitation falls as rain, and most rain falls on oceans. The rain that falls on land and becomes freshwater is critical for our living needs. Our lives literally depend on it.
- Ocean to Atmosphere — Water rises from the ocean and becomes part of the atmosphere by being transformed from its liquid state to its gas form, known as water vapor. This happens through the process of evaporation.
- Cloud Formation: Water Vapor & Dew Point — There are a few notes on dew point in chapter 1, but it’s worth revisiting here. Water vapor is a huge part of weather, and there are molecules of water vapor hanging out in the atmosphere all over the place in humid environments (a lot of water vapor is what causes humidity). But you don’t see water vapor unless it becomes saturated. What happens for water vapor to become saturated? It means the air is holding as much water vapor as it possibly can. It is 100% maxed out. All things being equal, the higher the temperature of the air (e.g. 90 degrees), the more water vapor it can hold before becoming saturated compared to lower air temperatures (e.g. 20 degrees). This point of saturation, called the dew point, usually arrives when warm air containing water vapor rises then cools down to its dew point. When the air cools to its dew point, the water vapor condensates (condenses) into tiny water droplets or ice crystals and you start to see it in the form of clouds, fog, dew, and frozen dew. Long story short, “clouds form when air rises and cools to its dew point.”
- Why You See Your Breath In Cold Weather — The process outlined above regarding air rising in temperature and cooling down to its dew point to create clouds and fog is the same process that leads to us being able to “see” our breath while outside in cold weather. When the air temperature is cold, it is very dry and unable to hold as much water vapor before being saturated compared to when the air is warm. Therefore, the moist air filled with water vapor from your lungs that you exhale overwhelms the cold air and leads to saturation immediately. As the point above touched on, when the air reaches 100% saturation, water vapor condenses into clouds and fog. In this case, you’re creating a tiny cloud in front of your face with every breath you take.
- Quote (P. 71): “Also, this is the stuff your breath makes when the warm, moist air from your lungs hits the cold, dry air of the winter morning. It’s the addition of the water vapor that quickly saturates the cold air. You’re forming a tiny cloud in front of your face with every breath you take.”
- Cold Weather = Less Rain & Snow — It seems counterintuitive, but the colder the air, the less likely it is to snow or rain. This is because, as the previous bullets discussed, cold air is unable to hold as much water vapor (i.e. moisture) as warm air. In other words, the air is dryer. When it is 95 degrees outside, that warm air can hold a lot more water vapor than the air outside on a 5-degree day. The ideal temperature for snow is 30-35 degrees because the air is cold enough for snow to form but also warm enough to hold a decent amount of water vapor. A temperature of 5 degrees is typically too cold to hold enough water vapor to create snow. This is also why there are fewer clouds and rain on really cold days; the cold air just isn’t able to hold enough water vapor to create these events. Overall, really cold days tend to be pretty clear.
- Water Vapor = Most Important Substance in Weather — Water vapor, or moisture, is the single most important substance in the air when it comes to creating weather. Without water vapor, there is rarely any bad or intense weather — and there is no humidity. It is the moisture from the ocean that starts most storms. The water vapor from the ocean rises, cools to its dew point, and condenses into rain drops and ice crystals that form clouds. These storms then blow towards land, and the clouds dump their contents of rain and snow. When the ocean gets nice and warm in the Tropics near the Equator, hurricanes can develop. Areas like Arizona that are far away from any body of water tend to have clear weather (except haboobs, which involve water vapor). Bottom line — water vapor must be present for storms, rain, and snow to appear. Temperature extremes are a different story — that has more to do with the fact that land is heated by the Sun very quickly compared to the ocean, then cools quickly overnight. Places near the ocean tend to have more moderate temperatures by comparison.
- Quote (P. 56): “If there is one substance in the atmosphere more involved with weather than any other, it is the gaseous form of water. At its most concentrated, water vapor makes up only 4 percent of the atmosphere, and yet, almost no important weather takes place without it. Without water vapor to condense into droplets of water or ice crystals as air rises and cools, no clouds would form in the sky. Without water vapor, there would be no precipitation — no rain and no snow. The cycling of water through the environment, as described in Chapter 3, would come to a screeching halt without water vapor in the atmosphere.”
- Chapter Takeaway — Water plays a major role in weather. It starts with ocean water evaporating into the atmosphere, where the warm air containing water vapor cools and condensates, creating clouds, rain, and snow. The rain and snow then fall to the surface, where the water makes its way back to the ocean. Sometimes the journey back to the ocean is quick; other times it can take many years. Clouds are formed when air rises up, increasing in temperature, then cools down to its dew point. At this point, the water vapor in the air condenses into tiny water droplets or ice crystals, creating clouds. Warm air can hold more water vapor than cold air, which is why hot days are more humid than cold days.
Ch. 4: Blowing In the Winds
- Understanding Air Pressure: Part I — Pressure is the force exerted by air due to the weight of the atmosphere pressing down. Air is a gas, so its weight is always being exerted in all directions. If you hold your arm straight out, it will hang there without a problem, despite the fact that air weighs 14 pounds per square inch and is pressing down on you. The reason your arm doesn’t collapse under that weight is that there is equal pressure being exerted from the ground up, pushing up from underneath your arm.
- Understanding Air Pressure: Part II — When you see a number presented for “pressure,” it’s giving a measure of how many molecules are stacked up in a column of air directly overhead pressing down on us. Air pressure changes from top to bottom, and the air pressure being generated at any given spot on the planet can be different. Air pressure is greatest at the bottom of the column (i.e. lower altitudes) because there are more air molecules stacked up and pressing down. At the bottom in lower altitudes, air molecules are also more bunched together, which is why breathing in oxygen is not a problem. As you go higher up in that column of air (i.e. higher altitudes), the air pressure decreases because there are fewer air molecules stacked above and pressing down from overhead. This means that air molecules, and therefore oxygen, are more spread out in higher altitudes. This is why it is harder to breathe in higher altitudes and why we need pressurized cabins in airplanes; there is not enough pressure at higher altitudes, meaning air molecules are spread out and there isn’t enough oxygen to breathe comfortably.
- Quote (P. 86): “At a particular place on the surface, pressure is a measure of how many molecules are in a column of air directly overhead. The air pressure changes from the bottom to the top. The force is greatest at the bottom because all the molecules are overhead. The higher up the column, the less the air pressure, because fewer air molecules are always overhead. At about 18,000 feet, about three and a half miles up, about half of the atmosphere’s molecules are overhead and half are below you. So the air pressure is about half what it is at the surface. Feeling the downward force of all of that atmosphere overhead, air molecules congregate more closely to one another near the ground than up in the sky.”
- Quote (P. 87): “Vertically, or up and down, the differences in air pressure are huge compared to the differences horizontally, or sideways. In fact, the bulk of the air is so concentrated near the ground that a trip up an elevator in a skyscraper carries a passenger through greater differences in air pressure than would be measured across 1,000 miles of the surface.”
- Wind & Air Pressure — Wind is caused by differences in air pressure. When there is a difference in pressure between two areas, air moves from the higher-pressure area to the lower-pressure area, creating wind. The atmosphere is constantly trying to balance out these pressure differences, which leads to wind. Larger pressure differences can also cause storms, hurricanes, tornadoes, and other weather events as the atmosphere works to restore balance.
- Wind: Earth’s Rotation — Another factor that influences wind is the Earth’s rotation, which causes winds to bend and change direction due to the Coriolis Effect. This effect (i.e. Earth’s rotation) is also responsible for the jet stream, a really fast-flowing air current way up above us that makes flights from west to east quicker than those traveling east to west. These winds can reach speeds up to 100-200 miles per hour. No wonder flights from the west coast to the east coast are so much faster!
- High Pressure & Low Pressure Systems — You hear these two systems referenced by weathermen a lot. In short, high pressure systems create clear skies and light winds, while low pressure systems create clouds, storms, and rain. During a high pressure system, air in the jet stream converges together so tight together that it must sink. When it sinks, it sinks in a clockwise direction and begins to warm up as it gets closer to the ground. This warming of air is why there are no clouds and the weather is nice. Remember, it’s when warm air rises, then cools to its dew point that clouds and storms can form. And that’s exactly what happens in a low pressure system. In this system, the air from a high pressure area leaves and enters the low pressure system. The air in a low pressure system rotates counter-clockwise until it reaches the center of the system. At this point, it rises up into the atmosphere to fill gaps in the jet stream above the system. As the warm air containing water vapor rises up, it eventually cools down to its dew point and condenses, creating clouds and storms.
- Chapter Takeaway — High pressure systems produce clear skies and calm conditions. Low pressure systems produce clouds, rain, and storms. This is because, during high pressure systems, air from the jet stream is sinking down to the ground and heating up as it goes, while in a low pressure system, warm air from the surface is moving up into the atmosphere, where it cools to its dew point, condenses, and creates clouds, rain, and storms. Warm air contains more water vapor than cold air, which is why it’s the process of warm air cooling that causes these effects.
Ch. 5: Getting Cirrus
- Making Clouds & Storms — This process has been discussed several times throughout the book already, but it’s worth revising to cement the material. Clouds form when the water vapor in warm air (which always contains more water vapor than cold air) rises, cools down to its dew point, and condensates (condenses) into rain drops and ice crystals that take the shape of clouds. To reiterate, the dew point is the temperature at which the air is 100% full of water vapor (i.e. “saturated”). Cold air cannot contain as much water vapor as warm air before becoming saturated. This is why, when warm air containing water vapor rises into the cold air above, it eventually cools down to the dew point. When the dew point is reached, condensation occurs as the water vapor condenses into rain drops and ice crystals. This condensation process creates clouds that can cause rain, snow, and stormy weather.
- Quote (P. 102): “If you are going to make a cloud, you are going to have to cool air to its dewpoint — to the temperature where it is saturated with water vapor, and the vapor begins condensing into ice crystals or water droplets. That’s what a cloud is — ice crystals or tiny water droplets, or a combination of both. The best way to cool air is to get it off the ground and move it up into the realm of lower air pressure, where it expands and its temperature drops. One way or another, you’re going to have to lift the air.”
- Quote (P. 106): “Making a cloud without getting air to rise is hard, but something else is even more essential. The most important thing that has to happen for a cloud to form is for the temperature of the air to fall to its dewpoint or frost point — that condition called saturation, when it holds all of the water vapor that it can and not one molecule more. As it cools beyond this point, it causes condensation, and a cloud forms.”
- Clouds & Storms: The Shower Example — A very exaggerated and simplistic analogy for how clouds and storms are created happens in the shower every day. When you take a shower, the warm water that is being released creates evaporation. The warm air rises to the ceiling and cools to its dew point. The water vapor then condenses into little water droplets and creates a “mini cloud” of visible steam. If the shower is left on long enough, the steam will cause condensation where water drips on surfaces like the mirror and walls. This scenario is a very simplistic and exaggerated version of what actually happens in the atmosphere, but you are literally “making it rain” in the bathroom. This process happens faster when the shower room is cold (like in the winter) vs. when the room is warm because cool air cannot hold as much water vapor as warm air. This means the water vapor from the shower saturates the cold shower room air faster, creating steam faster.
- Quote (P. 24): “Water vapor is an invisible gas, but you see and feel the effects of the air’s relative humidity all of the time. When you take a shower, for example, you’re adding so much water vapor to the air that it is quickly saturated. Condensation is producing tiny water droplets and you’re standing in a little cloud. Step out of the shower, and you might begin to feel a little chill. You are stepping into air that is less humid, and heat is leaving your wet skin through evaporation. The heat is used to convert the liquid into the water vapor that is being absorbed into the air. The bathroom mirror is fogged up because the air up against its cooler surface has fallen to its saturation point and some of its water vapor has condensed into dew. Clouds form when air rises and cools to its point of saturation.”
- Why Does Arizona Have Less Rain? — Why does a state like Arizona experience very little rain despite having a very warm climate? There are a couple reasons, and they tie into the material covered in this book. For one, our air, although it is very warm, is also very dry. We don’t have air masses full of water vapor from an ocean pushing into us like California and Florida do. We don’t live close to a large body of water. As a result, our air generally contains very little water vapor, and water vapor is a huge driver of storms and weather events. Second, Arizona sits in a region of high pressure systems. These high pressure systems are sending air from the jet stream down to the ground, where it warms up, dries out, and produces clear skies and good weather. In contrast, low pressure systems send warm air up, where it cools and condenses into clouds, rain, and storms.
- Why Mountain Areas Are Stormier — When air encounters a mountain that it can’t get around, it rides up its slopes, which gives it a little boost of a lift that’s called an orographic lift. This causes the air to rise, cool to its dew point, condensate into rain drops and ice crystals, leading to clouds and stormy weather. This is partly why places like Colorado, the Sierra Nevada, and other mountain areas experience cooler, rainier weather.
- “Unstable” Air: Upper Atmosphere Temperature Matters — The temperature of the air in the upper atmosphere plays a crucial role in cloud and storm formation. Rising air cools at a set, constant rate. But depending on the temperature of the air that it is rising up into, there may be more or less of a chance of clouds and rain developing. When the air above is much cooler than the warm rising air (i.e. “unstable”), it remains warmer than the surrounding air, so it keeps rising and rising. The higher it can rise, the more of its water vapor will condense into clouds, and the taller and stronger the clouds can grow. The stronger and more vertical the cloud, the more likelihood of rain and storms. This is why meteorologists are very interested in finding out the temperature in the upper atmosphere and it’s why we release weather balloons twice per day. If we know the temperature in the upper atmosphere, we can compare it to the set rate of cooling of warm rising air and determine the likelihood of rain and storms developing.
- Types of Clouds — Clouds are labeled according to their appearance and altitude, but there are really only four main types of clouds: stringy, heapy, layered, and vertical. And there are only three main types of altitudes that clouds develop in: high, middle, low. The types of clouds are provided below, along with a few photos:
- High Altitude Clouds:
- Cirrus — Common in dry areas like Arizona. Very thin, wispy clouds. These clouds are made of ice crystals that have fallen from a parent cloud. These clouds could mean bad weather is on the way.
- Cirrocumulus — Thin, rippling clouds that look like fish scales. Could signal rain coming soon.
- Cirrostratus — Very thin and sheet-like, usually spread over the entire sky. If these clouds thicken, rain or snow might be coming. These clouds often signal an oncoming warm front.
- Middle Altitude Clouds
- Altocumulus — Rarely cause rain that reaches the ground. Could signal an oncoming cold front.
- Altostratus — Similar to cirrostratus but are thicker. These usually arrive ahead of widespread rain or snow.
- Low Altitude Clouds
- Stratocumulus — Thick, gray, patchy clouds. Appear in rows and patches. These look threatening but rarely bring rain.
- Stratus — This is basically fog. Not a rain cloud but can bring mist and drizzle.
- Nimbostratus — These are true rain clouds. Dark gray clouds that cover much of the sky and block out the Sun. These deliver consistent rain.
- Vertical Clouds
- Cumulus — The more vertical a cloud develops up and down, the stronger it is. Cumulus clouds are big, bright, puffy white pillows with dark undersides. Their tops look like cauliflower. They usually appear in partly cloudy weather. In warm, humid, unstable conditions, “cumulus congestus” can develop. These clouds can quickly stack on top of each other and bring scattered showers. If they continue to build, they can develop into giant cumulonimbus clouds (next bullet).
- Cumulonimbus — These are enormous, towering clouds. They can form individual storms or be part of a line of towers called a “squall line.” These clouds can bring lightning and downpour of rain and hail. They can also spawn tornadoes. The biggest of these monster clouds is called a “supercell” and can bring really serious weather violence.
- High Altitude Clouds:
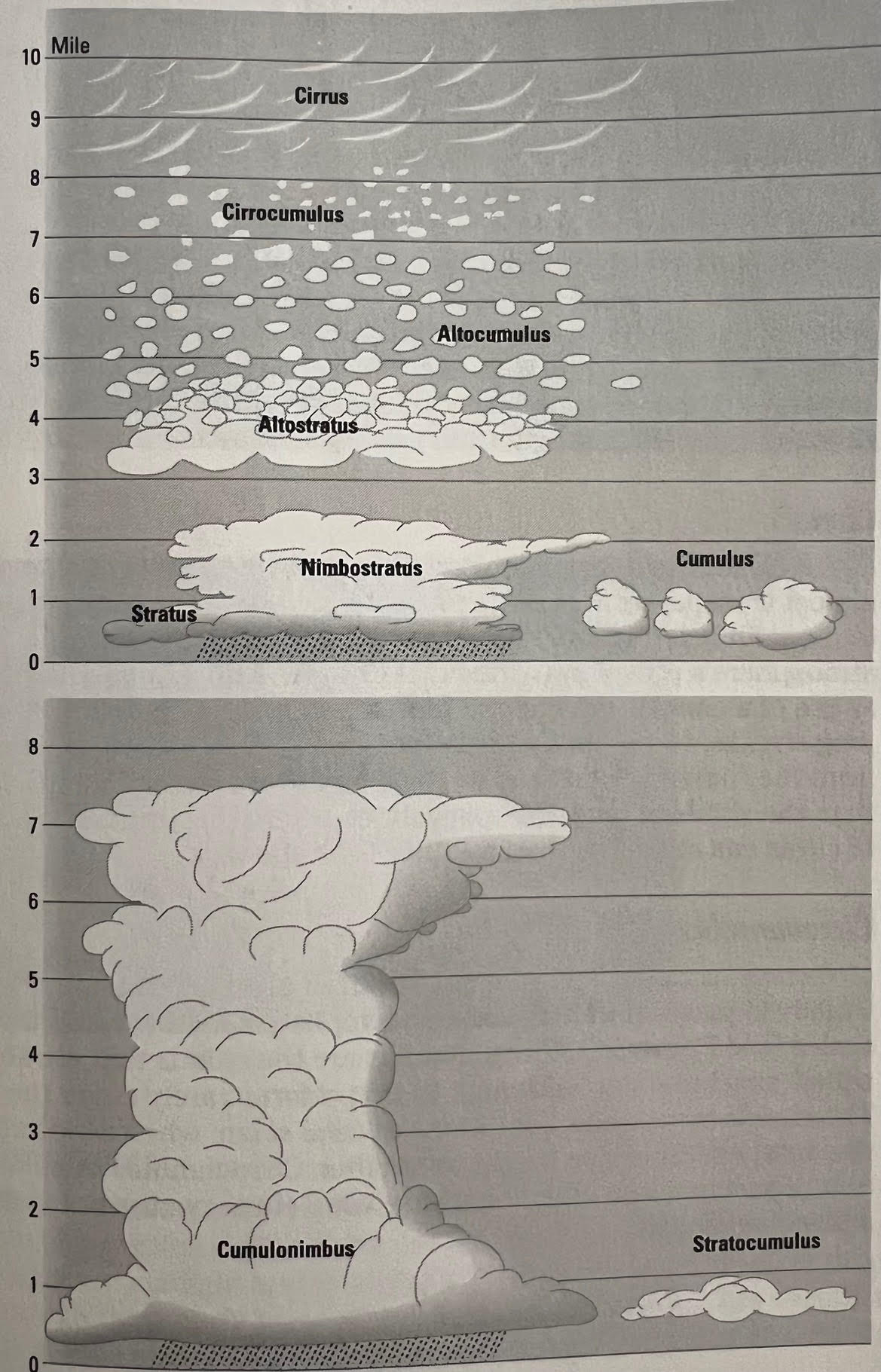
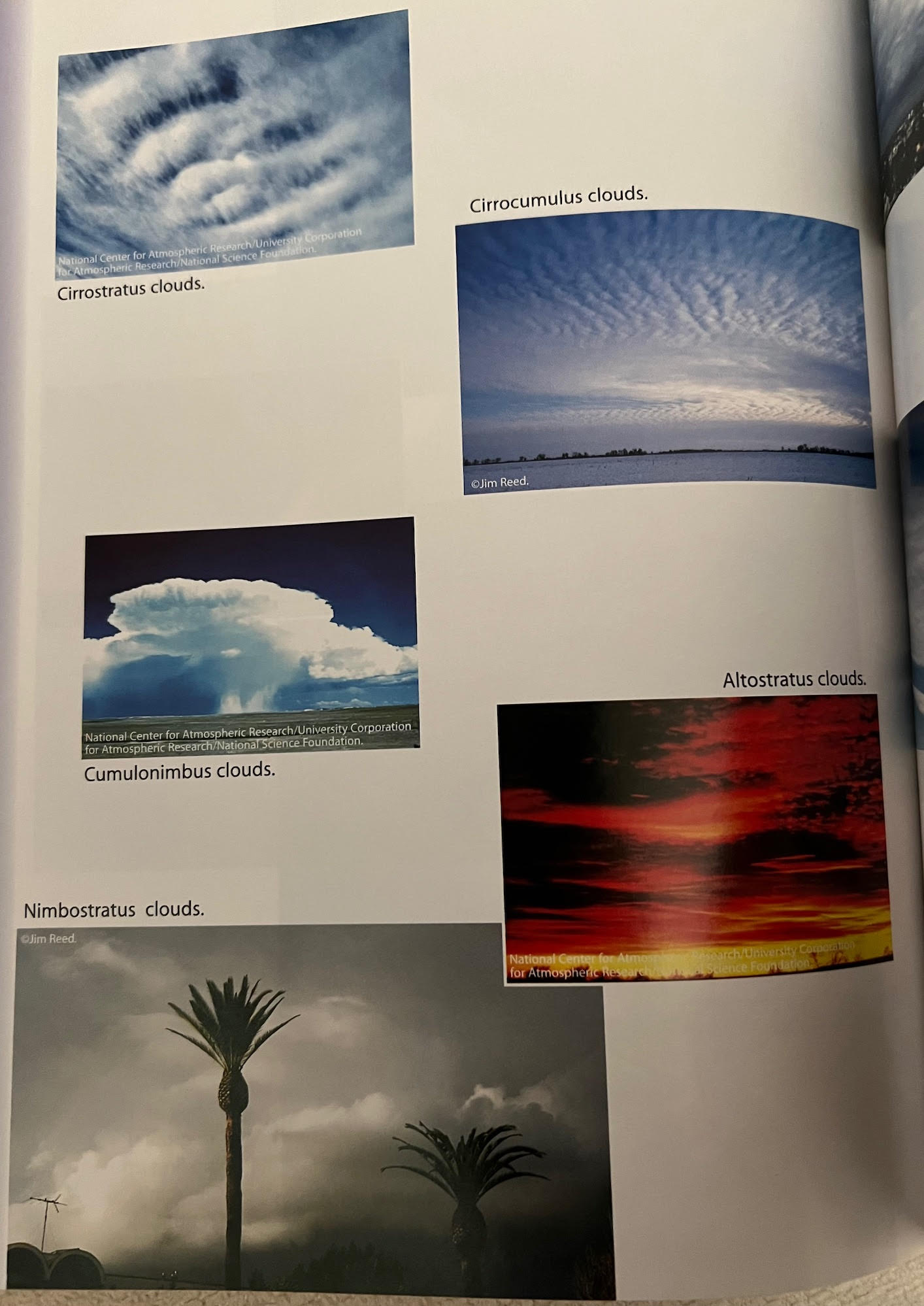
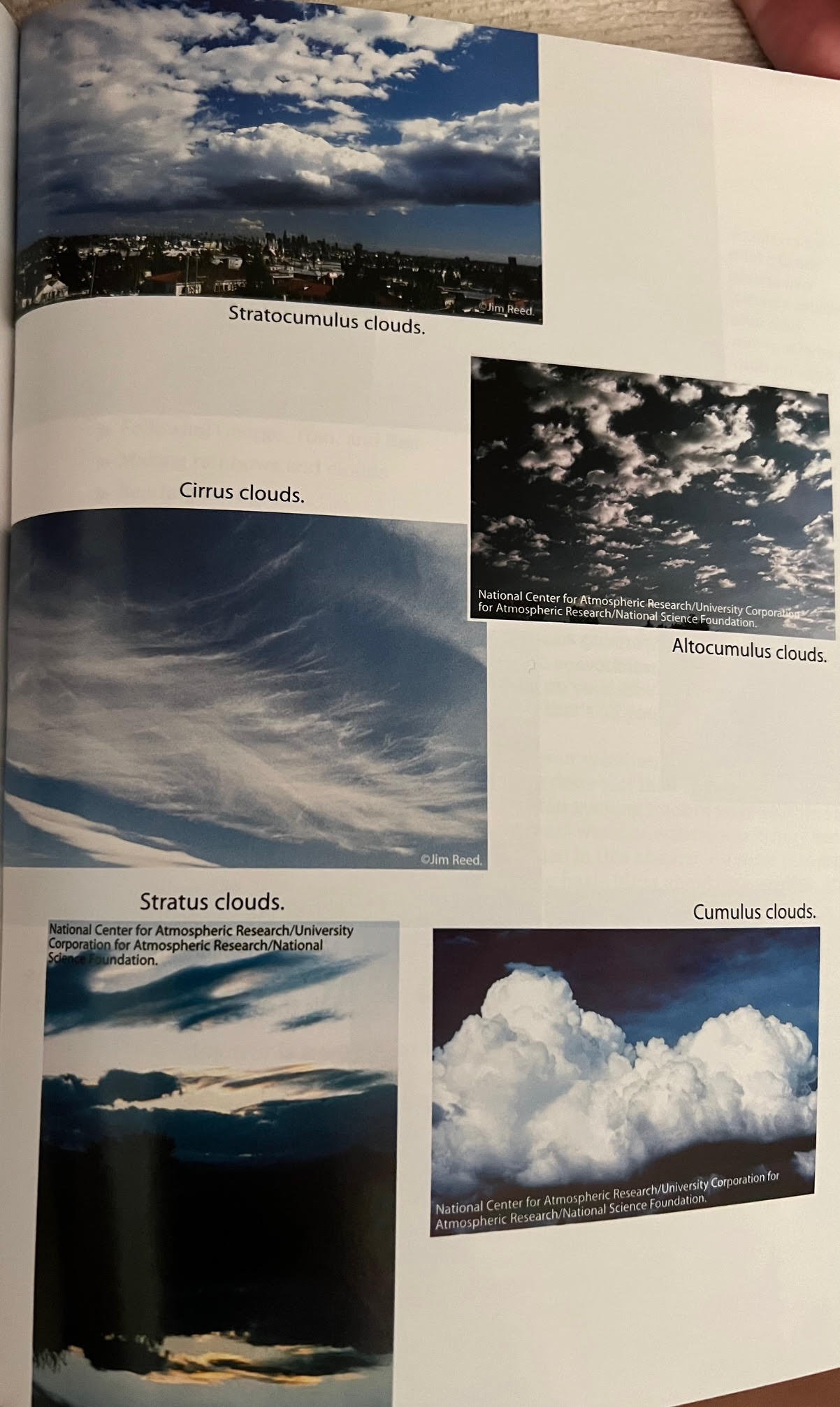
- Chapter Takeaway — There are many types of clouds that form at high, medium, and low altitudes. Generally, the taller and more vertically developed a cloud is, the more intense the weather it can produce. Tall clouds, like cumulonimbus clouds, develop when the air in the upper atmosphere is significantly colder than the rising warm air, allowing the warm air to continue rising and condensing, building the cloud higher. This vertical growth can lead to more intense weather phenomena like thunderstorms, heavy rain, hail, and sometimes tornadoes.
Ch. 6: Climate Is What You Expect; Weather Is What You Get
- Climate vs. Weather — The key difference between climate and weather is time frame. Weather is the day-to-day action occurring in the atmosphere. Climate is the pattern of weather that a region experiences over years and decades. Observing climate conditions helps scientists figure out answers to big picture questions about our planet. A region’s climate is classified according to two features: (i) the average amount of precipitation — rain or snow — that falls during the year and the various seasons; and (ii) the range of average high and low temperatures during a typical day and the seasons. Below are the basic climate types:
- Tropical Climate — The regions around the Equator experience a tropical climate. This area gets the most sunshine, as the Sun is high in the sky all year long. It’s always warm, and often there is a lot of humidity and rain thanks to the oceans nearby.
- Dry Climates — The deserts of the world experience a dry climate. The Southwestern U.S. region is an example.
- Mid-Latitude Mild-Winter Climates — The Southeast U.S., Northern California, and Pacific Northwest experience this climate. The nearby oceans contribute to some humid and rainy conditions in these areas, but all three regions have mild winters.
- Mid-Latitude Severe-Winter Climates — These regions are located further north of the mild-winter climates. Think of areas like the Northeast and Midwest. The cold climates these regions experience are largely due to the cold polar air masses that develop over Canada and Alaska. These polar air masses often move south, bringing cold temperatures in the winter.
- Polar Climates — Winters are very long and severe in these climates. Due to their location near the north and south poles, the Sun never really hits these areas directly like it does for the tropics near the Equator. The Sun hits these areas more at a glancing, low angle, so the land never really gets very warm. In the winter, the Sun often doesn’t rise at all, which only contributes to the severe cold. Northern Canada, Alaska, and Antarctica experience this climate.
- What Makes Climates Different? — We understand that there are different climates around the world. But what makes them different from each other? There is a combination of factors, but they all center around the fact that the Sun heats the world unevenly, and the planet’s moisture is unevenly distributed. If these two things were distributed evenly across the planet, we would have one uniform climate. Below are a few key factors that drive climate:
- Sunshine — The difference in climate between the north and south poles and the Tropics near the Equator is evidence that more sunshine means a warmer climate. The Sun causes a lot of heat at the Equator because its energy is coming down in a more direct manner compared to the glancing angle that the poles experience.
- Land & Water — About 50% of the Sun’s energy passes through the atmosphere and hits the surface (i.e. land and water). Land heats up and cools down much faster than water, creating more temperature extremes. Huge land masses like North America create very hot and cold temperatures because the Sun’s energy warms the ground and the air above it and is then redirected back up to the atmosphere. And at night, the heat absorbed by the land cools rapidly. It’s different for the oceans. Oceans absorb the Sun’s heat and hold onto it for a lot longer. This helps explain desert climates, where the land is absorbing the Sun’s heat and there are usually no bodies of water close by. It also explains why places like Florida have more moderate temperatures year-round; there’s more ocean nearby to absorb some of the Sun’s heat.
- Oceans — Oceans send water vapor up into the air via evaporation. As it rises, this air will cool to its dew point, condense into rain drops and ice crystals, and release. As a result, areas that are located near any of the oceans (e.g. Florida and California) will experience climates that are often more humid and stormy.
- Mountains — Mountain towns generally experience cooler and stormier weather because air that can’t get around the mountain rises up its slopes, cools to its dew point, condenses into rain drops and ice crystals, and is released by the clouds that are created. This causes a lot of snow and rain. A town on the other side of the mountain can experience better weather because that air that rose on the front side falls on the backside, creating better weather conditions.
- Quote (P. 127): “Air flowing into the side of a mountain has the same effect as water in a river flowing up against a large rock. It rises up and over the mountain. As it does this, the rising air cools, causing clouds to form. Often it snows or rains. On the other side of the mountain, something very different happens. The air that has been dried out on the way up one side now falls back down the slope and warms up again. The climate on the side where the wind comes often is cool and wet. On the other side, in what is called the rain shadow, the climate is dry.”
- Elevation — Air cools as it rises. Why? Because at higher elevations there is less air pressure, which means there are fewer air molecules stacked on top of each other and pressing down. This means the rising air has room to expand rather than being bunched up like it is at sea level (see Ch. 4 notes for more). This expansion allows the air to cool. This helps explain why places in higher elevations (e.g. Papa and Irma’s cabin; Flagstaff, AZ; Denver, CO) experience cooler temperatures.
- Key Takeaway: Atmosphere Responds to What’s Underneath — One of the big takeaways from this book is that, in many ways, weather begins with what’s happening at the surface. The atmosphere responds in a big way to what’s going on underneath. And because 70% of the planet is covered by water, the ocean is a major, major contributor to weather and climate. This fact helps explain why a place like Seattle gets so much rain. In the Pacific Ocean, big storms are being created by water evaporating/rising up, cooling to its dew point, and creating clouds of rain drops and ice crystals. Winds often blow these clouds and storms northwest into regions like Seattle, contributing to the rainy climate there. The mountains in the Northwest also contribute. Similar events are happening in the Atlantic Ocean, causing storms and hurricanes in Florida. Places like Arizona, meanwhile, don’t have any large bodies of water nearby creating and pushing storms into the area. This plus the fact that the state is located in a consistently high pressure system area contribute to the region’s very dry air and clear weather.
- Quote (P. 129): “Here is a big idea in weather science: The atmosphere responds in a big way to what’s going on underneath it. When it flows over ice sheets and snow fields, for example, it gets the chills. When it flows over the continents, it picks up some of the heat or some of the cold of the land. But remember this large fact about your favorite planet: Earth is covered with water more than anything else — 70.8 percent, to be exact — and the ocean is where a lot of the weather and climate action is. In fact, the sea and the sky influence each other so strongly that weather scientists have come to refer to them as parts of a single coupled system. Get this idea down and mention it as often as you can.”
- El Niño — Every 3-7 years, El Niño comes along in the winter months. El Niño occurs when unusually warm temperatures emerge in the surface waters in the central and eastern Pacific Ocean, near the Equator. Because the ocean and atmosphere are so interconnected, this warming disrupts typical weather patterns across the globe. During El Niño events, trade winds that normally blow from east to west weaken or even reverse, allowing warmer water to spread eastward. This leads to unusually high amounts of rainfall in regions like the Southwest U.S., while regions like Southeast Asia and Australia experience drought. These disruptions can lead to floods, droughts, and weird shifts in temperatures during the winter. The storms themselves aren’t all that dangerous; it’s the fact that El Niño causes so much unusual weather in places that aren’t used to these conditions that is dangerous. For example, the Southwest U.S. usually experiences huge amounts of rainfall during an El Niño, and the desert climate easily morphs into massive floods in those conditions. In other places that are used to rainfall, the droughts caused by El Niño cause issues.
- La Niña — The sister of El Niño is La Niña. Opposite of El Niño, La Niña is characterized by cooler-than-average ocean surface temperatures in the central and eastern Pacific Ocean near the Equator. It happens when trade winds strengthen, pushing warm water further west and allowing cold, deep ocean water to emerge along the Pacific coasts of the Americas. This cooling effect disrupts global weather patterns in ways opposite to El Niño. Unlike El Niño, which causes opposite weather to happen in many locations, La Niña typically exaggerates the existing climate; for example, it brings even drier conditions to the Southwest U.S. and even wetter, stormier weather to the Pacific Northwest and northern regions of the U.S.
- Quote (P. 136): “Here is a rule that is not always exactly true, but still is useful to compare the impacts of El Niño and his contrary sister. Where El Niño is warm, La Niña is cool [temperature of ocean water]. Where El Niño is wet, La Niña is dry. While El Niño conditions and their seasonal impacts look very different from normal, La Niña conditions often bring winters that are typical — only more so.”
- We Live In an Ice Age — The first ancient humans emerged about 2.5 million years ago. Climate scientists agree that an ice age has been going on for the last 2.6 million years, and we’re still in it right now, albeit we’re living in the “warming phase” of the ice age. This means that humans have only ever lived in an ice age. The glaciers and ice sheets at the north and south poles are evidence of that. Indeed, for hundreds of millions of years in the distant past — before humans and in the age of the dinosaurs — the Earth was often 14-25 degrees warmer than it is today. It’s true that the last century or so has seen average temperatures rise consistently, but we are still not very close to how hot Earth used to be during certain stretches of time. The main concern today is how fast temperatures are climbing year-over-year and whether humans are causing that.
- Quote (P. 139): “Did you know that for long periods of time the Earth’s climate was a lot warmer than it is today? Some researchers estimate that as little as 10 percent of Earth’s past has been ice ages. Others think it’s longer than that. But still, researchers agree that for hundreds of millions of years it was between 14 degrees and 25 degrees warmer than now. For example, one of those warm periods was about 65 million years ago — about the time the dinosaurs became extinct.”
- Chapter Takeaway — It seems backwards, but the atmosphere, and therefore the weather and climate, really depends on what’s going on underneath it. The land and water on the surface are what mostly influence the weather above. For example, ocean water evaporates, creating rising air that condenses to its dew point and leads to rain drops and ice crystals that form clouds. These clouds get blown around by the jet stream and deposit rain and snow in certain regions. Land absorbs and reflects the Sun’s energy, creating really warm and cold temperatures compared to places located near the ocean, which absorbs and retains the Sun’s energy for a lot longer than land, creating more moderate temperatures.
Ch. 7: The Greatest Storms on Earth
- Hurricanes — Hurricanes are the greatest storms on Earth. These are essentially giant tropical storms that band together, rotate around a center point, and are fueled by extreme winds — hurricanes reach wind speeds of at least 74 miles per hour, although many of them top 100 miles per hour. The hurricanes that affect the U.S. form every year in the Atlantic Ocean, the Gulf of Mexico, and the Caribbean Sea before blowing towards the states in the Gulf Coast and the East Coast. June through October is considered hurricane season, as this is the time of year when the water in the Atlantic Ocean north of the Equator is at its warmest. It’s this warm water that births the storms that later become a hurricane. Cold water is not as effective at creating and fueling hurricanes, which is partly why the West Coast doesn’t experience them; the Pacific Ocean is a little cooler.
- Quote (P. 143): “Not for nothing are they called the greatest storms on Earth. Few things in nature are more powerful than hurricanes. The giant tropical storms that come swirling out of the warm waters near the Equator like enormous coiled springs wreak death and destruction across the world every year. In an average year, 85 tropical storms form around the world, including 45 which will reach the wind intensity of at least 74 miles per hour, and so be classified as hurricanes.”
- Quote (P. 144): “A hurricane is a tropical storm run amok — a rotating mass of thunderstorms that has become highly organized into circular cells that are ventilated by bands of roaring winds.”
- Quote (P. 144): “In the North Atlantic, the hurricane season that threatens the Gulf Coast and the East Coast of the United States begins in June and continues through the end of October. This is the time of year when the water in the Atlantic Ocean north of the Equator is at its warmest. By October, the water in the region is beginning to feel the effects of autumn as the Sun’s direct rays shift toward the ocean south of the Equator.”
- Birth of a Hurricane — How is a hurricane formed? For hurricanes that affect the U.S., the process often starts in the warm waters of the Tropics near the Equator, where the Sun beats down and keeps the ocean’s surface around 80 degrees or warmer. This warm water, aided by low pressure in the area, is always ready to evaporate. Winds are light, so air can rise. Here, the warm water vapor rises, cools to its dew point, and condenses into rain drops and ice crystals that make up thunderclouds. After several of these clouds are created, they begin to cluster and build some counterclockwise spin thanks to the wind and the Coriolis Effect (i.e. Earth’s rotation). A system of low pressure develops at the very center. The storm continues to grow bigger and spin faster as it gains energy and more water vapor from the warm ocean. Interestingly, the center, the eye, is clear, does not have any clouds, and winds are fairly calm. Outside of the eye, the storm continues to gain momentum and becomes classified as a hurricane after reaching 74 mph wind speeds.
- Features of a Hurricane — Once a hurricane reaches land (e.g. Florida), it can become deadly. Although the wind is often thought of as the deadliest part of a hurricane, the mass flooding it brings has proven to be more dangerous to people living along the Gulf Coast. Hurricane Katrina, which tore through and flooded New Orleans in 2005, caused more than 1,300 deaths and $125 billion in damages. Below are a few ways hurricanes cause damage once they reach shore:
- Storm Surge — The greatest threat to property and people is the “storm surge,” a 50-100-mile-wide dome of water that sweeps up and over the shoreline where the hurricane comes ashore. This surge of water, which is almost like a mini tsunami, overwhelms the area, flooding houses and businesses.
- Winds — Hurricanes often reach wind speeds well north of 110 miles per hour. You do not want to be anywhere near it when it comes ashore. These winds can extend outward from the eye for 25 miles for small hurricanes and 150 miles for large hurricanes. This means you will feel the hurricane’s wind well before it arrives.
- Tornadoes — A hurricane can spawn tornadoes that rip through the area well after the big storm has passed through.
- Flooding Rainfall — Hurricanes dump huge amounts of rain on the area (6-12 inches), often producing severe flooding. In fact, flooding, not wind, has been the main cause of hurricane-related deaths in the U.S.
- Running Out of Gas — Once a hurricane hits land, it usually begins to run out of steam. This is because the warm water in the ocean is the hurricane’s fuel source. No warm water to suck up, no hurricane.
- Quote (P. 155): “When a hurricane moves over land, usually it marks the beginning of the end for the big storm, because it is cutting itself off from its fuel supply — the warm water of the ocean. Unless it crosses back over warm ocean water at some point, eventually it will run out of gas.”
- Forecasting Hurricanes: Zig & Zag — The problem meteorologists have when it comes to identifying exactly where a hurricane will hit is that these giant storms don’t follow a straight, predictable route in the ocean. They zig and zag all over the place. They stop and start. This unpredictable pace and pattern of movement makes it really hard for meteorologists to know who needs to pack up and leave immediately and who is outside of the impact area. As far as rating a hurricane — they are classified based on wind speed, even though the storm surge and flooding they cause are equally dangerous. Below is the scale:
- Category 1 — 74-95 mph
- Category 2 — 96-110 mph
- Category 3 — 111-130 mph
- Category 4 — 131-155 mph
- Category 5 — 155+ mph
- Chapter Takeaway — Hurricanes start as tropical storms that typically emerge out of the warm waters of the Atlantic Ocean and Gulf of Mexico. The warm water surface temperatures there cause the water to quickly evaporate, where the water vapor rises, cools to its dew point, and forms into rain drops and water crystals that make up storm clouds. As these storm clouds grow and cluster together, the system can intensify into a hurricane (wind speeds of 74+ mph). The hurricane continues to strengthen as long as it remains over warm water, which is its fuel source.
Ch. 8: The Ways of Winter
- Defining Winter — Although everybody feels the weather effects of winter well before it, the official first day of winter is December 21. This is the “shortest day” of the year, meaning it’s the day that the Northern Hemisphere receives the least amount of energy from the Sun. We receive the fewest hours of sunshine on this day. The “longest day” of the year is June 21, which is the day we receive the most amount of energy and sunshine from the Sun. Again, going back to notes from chapter 2, the reason we even have seasons like winter is the Earth’s 23.5-degree tilt. From June 21-December 21, the Sun’s arc slowly moves further and further South. From December 21-June 21, it slowly moves north towards us.
- Quote (P. 168): “On December 21 or 22, the Sun’s heat energy reaching the lands and waters of the Northern Hemisphere is at its lowest of the year. Often, this is referred to as the ‘shortest day’, meaning not that it has fewer hours, of course, but that it has fewer hours of sunlight than any other day. Since June 21, the arc of its daily pass through the sky has been dropping farther and farther toward the southern horizon, and on this day, the ‘winter solstice’, it stops.”
- Cold Air Marches South — Cold temperatures are a staple of winter. The reason it gets so cold has to do with the polar air masses of the north in Canada and Alaska creeping down south toward us in the middle latitudes. These air masses bring cold air, and they run up against existing warmer air masses. The collisions between these cold and warm fronts create the storms of winter. Because these cold polar air masses come from the north of us and creep south, the northern states in the U.S., like Minnesota, and the Midwest states get hit first and experience the coldest and longest winters in the country. This is also partly why the Southwest states like Arizona and Texas have milder temperatures in the winter; it takes the polar air masses longer to get there and they aren’t as cold by that time.
- Quote (P. 169): “Winter is when it gets cold. As the arc of the Sun has slanted farther and farther south, the polar air masses have been migrating deeper and deeper down over the Northern Hemisphere’s middle latitudes, where most people live. This migration of cold air is what makes winter what it is: a battle of warm and cold air masses. The battleground stretches clear across the country. What you feel in your bones is the fact that for quite some time there, the cold air has seemed to be winning every battle, gaining more and more territory over the warm.”
- Quote (P. 172): “By about the third week of January, on average, the cold air and its jet stream and the storms have migrated as far south as they are going to go. These are generally the coldest days of the season and often are the stormiest.”
- Quote (P. 174): “During the months of the Northern Hemisphere’s winter, the cold polar air masses and the warmer subtropical air masses to the south often tangle with each other over the middle latitudes. It is the time of greatest temperature contrasts in the upper atmosphere over this region because the polar air is plunging farther south toward the warm Equator than any other time of year.”
- Sun’s Uneven Distribution of Energy — This was mentioned in one of the early chapters, but the reason we have these warm and cold air masses that battle with each other and create winter storms described in the bullet above has to do with the Sun’s uneven distribution of energy. Imagine a house. One side of the house is filled with cool air and one side is filled with warm air. A similar thing is happening on Earth, as the Sun heats the Tropics at the Equator and the North and South poles experience very cold temperatures due to less direct sunlight. The atmosphere tries to balance the two sides by moving these air masses around. This movement causes warm and cold air masses to run into each other, creating storms. In the winter, as the bullet above describes, the cold air masses of the north move south into us as they make their way toward the warm Equator.
- Quote (P. 170): “After all, if it were not for this uneven distribution in the heat energy from the Sun between the Tropics and the polar regions, Weather For Dummies would be a pretty thin book. No other season brings out these differences as dramatically as winter.”
- Western U.S. Winter Weather — Because the West and Southwest regions of the U.S. are furthest away from the cold polar air masses marching down from the north, they experience milder winter weather. In fact, the weather experienced in these regions is more regulated by the storms blowing inland from the Pacific Ocean. For example, most rainy and cold winter weather that California experiences is a result of a Pacific Ocean storm blowing in from the west. On the other side, the winter weather that the Midwest and Eastern regions experience is mostly due to the cold polar air masses moving in from the north. This makes the northern and eastern parts of the country more susceptible to harsh winter conditions than the western regions. In the Southeast, the polar air masses don’t stretch far enough to really make an impact, and the Atlantic Ocean keeps the temperature pretty mild, which is why winters are nice in Florida.
- Quote (P. 170): “Between the western third of the United States and the eastern two-thirds is a world of difference when it comes to weather. Think of it this way: In the western third, winter weather is controlled by the prevailing westerly winds that come riding in from the Pacific Ocean. The key is the ocean. In the eastern two-thirds, winter weather is more often controlled by clashes over the middle of the country between the cold continental air masses from the north and the warm moisture from the Gulf of Mexico and the Atlantic. The key is the land.”
- Types of Winter Storms — The East Coast can experience harsh winter weather for several reasons, including the Coriolis Effect (i.e. Earth’s rotation) and the general west-to-east movement of weather systems. The jet stream, a fast-moving air current high in the atmosphere, generally flows from west to east across the U.S. due to Earth’s rotation. This means that many storms that develop in the central U.S. or even the Pacific Ocean can move eastward, eventually reaching the East Coast. Additionally, the East Coast can be influenced by cold polar air masses coming from the north and low-pressure systems moving up the coast, known as “nor’easters,” which can bring severe winter weather. Below are a few storms that are common in the winter:
- Nor’easter — A “nor’easter” is a storm that is born or rebuilt along the Atlantic Coast. Cold air clashes with the moist warm air of the Gulf Stream and the storm rides a northern track all the way up the East Coast. These are huge, powerful storms that bring huge amounts of rain, sleet, and snowfall. These storms often cause massive flooding. They are some of the worst winter storms we encounter.
- Pineapple Express — Once in a while, the polar jet stream that carries winter storms across the northern Pacific to the West Coast dips down south toward the tropical ocean near Hawaii. Here, these winter storms pick up some warm water vapor. The west-to-east winds then drag the warm moisture across the ocean, delivering especially heavy rains to the Pacific Coast. This kind of storm is called a “Pineapple Express” and brings huge rainfall and flooding to places California.
- Blizzards — These storms are some of the worst winter has to offer. They are characterized by really high winds, a ton of snow that blows all over the place because of the high winds, and freezing temperatures. For a “blizzard warning” to be issued, wind speeds need to hit at least 35 mph, the temperature must be 20 degrees or cooler, and these conditions must hold for at least three hours.
- Chapter Takeaway — For the West Coast, winter weather is primarily driven by what’s going on in the Pacific Ocean. Storms that develop out there often blow inland due to the natural west-to-east movement of the jet stream, bringing rain and snow. For regions like the Midwest and East Coast, the polar air masses that move south from Canada and Alaska make the winters very cold and stormy. These cold air masses clash with warmer air masses and create havoc.
Ch. 9: Twists and Turns of Spring
- Defining Spring — Spring is the transition between winter and summer, and it “officially” begins on or around March 21. On this first official day of spring, the Sun is directly over the Equator and there are exactly 12 hours of sunlight and 12 hours of darkness. In many regions around the country, spring brings highly unpredictable weather. Some days it brings nice weather, others it brings crazy storms. And some days it doesn’t show up at all and it feels like winter all over again. It’s also the season of thunderstorms and tornadoes; these storms are intense and wreak havoc in the Midwest.
- Quote (P. 196): “But spring has this difficult, fitful temperament. Some days it does great work, and you’re smelling the daffodils. The next day, it doesn’t show up, and you’re shoveling snow again.”
- Quote (P. 196): “Spring is the peak season for tornadoes, the most violent and terrifying winds on the planet.”
- Why Does Spring Bring Storms? — Thunderstorms and tornadoes are common in the spring because warm air masses, often from the Atlantic Ocean and the Gulf of Mexico, start moving north as temperatures rise. Think back to winter and the cold air masses from the north that move south into us — the opposite begins to happen in the spring. These warm, moist air masses clash with cold, dry air masses lingering from winter. The sharp contrast between the warm, humid air and cold, dry air creates the unstable atmospheric conditions needed for severe weather. The greater the temperature and humidity differences between these air masses, the more intense the storms that can result, leading to thunderstorms and, in some cases, tornadoes.
- Quote (P. 197): “In the Atlantic Ocean, the Bermuda High is beginning to gain strength and is shifting westward. Air circulating around it carries warm moisture from the Gulf of Mexico into the interior of the United States. The warm, jumpy moisture will clash with the cold, dry dense air masses left over from the continental winter. Cold and dry, warm and moist — air masses with these traits do not get along. The bigger their differences, the more severe the thunderstorms they make.”
- Redistributing the Sun’s Energy — Looking big picture, all storms and wind are the result of the atmosphere’s attempt to redistribute the Sun’s uneven heat energy and achieve balance across the planet. Looking at the spring season, the thunderstorms created during this period are the result of the Earth pumping water vapor from the warm ocean into the atmosphere so it can redistribute the warmth.
- Quote (P. 198): “Looking at the planet as a whole, thunderstorms are one of the main ways that Earth pumps water vapor from the warm ocean into the atmosphere and so begins redistributing the Sun’s uneven heat energy.”
- Creating Spring’s Thunderstorms — All storms require that warm air containing water vapor rises, cools to its dew point, condenses into rain drops and ice crystals, and forms into clouds. In the case of spring thunderstorms, the air that this warm moisture often from the Atlantic Ocean and Gulf of Mexico is rising up into is usually much cooler, which allows the air to keep rising and rising. This leads to those very vertical, towering cloud formations (cumulonimbus clouds) that produce intense thunderstorms and can lead to tornadoes. The bigger the difference between the temperature of the rising warm air and the cooler air above it, the bigger the storms. Because spring follows winter, the difference is usually pretty big.
- Quote (P. 199): “Like all storms, a thunderstorm needs rising air. . . And the temperature of the upper air needs to be arranged so that the air continues rising — conditions that weather scientists call unstable.”
- Lightning — Thunderstorms often produce lightning, big sparks of electricity in the sky. What happens is this: the giant cumulonimbus clouds that form a thunderstorm turn into a giant battery in the air; the movement of ice crystals and raindrops causes positive and negative charges to build up. The upper part of the cloud becomes positively charged, while the lower part becomes negatively charged. When the electrical charge difference becomes strong enough, lightning is produced. Most lightning bolts stay within the cloud or jump from one cloud to the next; only 1 in 5 bolts hit the ground. A single flash of lighting is five times hotter than the surface of the Sun.
- Quote (P. 210): “For some reason, a giant cumulonimbus thunderstorm cloud develops positive and negative electrical charges in different parts. It’s like a big battery. The ice crystals on top become positive, and the water droplets and rain at the bottom become negative. This strong negative voltage at the bottom of the cloud has a way of pushing the negative voltage on the ground out to the side of the storm, because like-voltages repel one another. So the ground beneath the negative bottom of the cloud is made positive. These different electrical charges grow stronger and stronger, and then ZAP! — a spark, a lightning bolt.”
- Quote (P. 211): “Four out of five lightning bolts are within the cloud of a thunderstorm or flash from one cloud to another. About one in five travel from a cloud to the ground, which is enough already. A stroke of lightning heats the air it strikes up to 54,000 degrees — roughly five times hotter than the surface of the Sun. Go figure.”
- Thunder — It should be “lightning and thunder” rather than “thunder and lighting.” When lighting strikes, it rapidly heats the air, causing it to expand explosively and create a shockwave, which we hear as thunder. Thunder happens at the same time as lighting, but sound waves move much slower than light waves, which is why we hear cracks of thunder after seeing lighting flash across the sky. This is also why thunder seems to stay around forever while lighting is so brief — it simply takes longer for the sound waves of thunder to reach us. The longer the delay between when you see lighting and when you hear thunder, the further away you are from the storm. If you experience an enormous crack — or thunderclap — that sounds like an explosion went off, you were obviously very close to where the bolt struck.
- Quote (P. 211): “Lightning strikes, and the air explodes, and the sound of the shockwave is thunder. The great boom lets go at the same instant as the lightning, but the sound waves travel so much slower than the light waves that the thunder always seems to follow the lightning.”
- Quote (P. 212): “Why does thunder seem to last so long while lightning is so brief? Again, it’s the speed of sound. It takes much longer for the thunder to reach you from the lightning stroke at the top of the cloud than from the streak you see near the ground.”
- Tornadoes — Tornadoes are nature’s most violent storms, even more so than hurricanes. Tornado wind speeds are capable of reaching upwards of 250 miles per hour. In fact, one of the most destructive tornadoes in history hit Oklahoma City in 1999 with winds of more than 315 mph. For a combination of reasons, conditions in the U.S. are ripe for tornadoes, especially in Tornado Alley across the Midwest. More than 1,200 tornadoes are reported in the U.S., far more than any other country. Like hurricanes, there is a scale that classifies tornadoes based on their wind speed. Below are the ratings:
- F0 — 40-72 mph
- F1 — 73-112 mph
- F2 — 113-157 mph
- F3 — 158-206 mph
- F4 — 207-260 mph
- F5 — 261-318
- How Do Tornadoes Form? — Tornadoes often form from massive thunderstorms called supercells. These intense storms feature rotating updrafts, and under the right conditions, tornadoes can “spin off” from these systems. While tornadoes can form in many places, Tornado Alley — a region including Texas, Oklahoma, Kansas, Nebraska, and Iowa — has ideal conditions. Why? Warm, moist air moving in from the Gulf of Mexico at lower levels, dry air moving in from the Rocky Mountains at mid-levels, and cooler air moving in from the Pacific Ocean or Canada at higher levels create the perfect storm for tornado development. The clash of these air masses, along with wind shear, contributes to the formation of tornadoes.
- Quote (P. 219): “These are the ingredients for the most severe thunderstorms and most powerful twisters — sharp differences in temperatures at different levels, big contrasts in dryness and moisture, and layers of powerful winds that are blowing from different directions at different speeds.”
- Chapter Takeaway — Spring is characterized by highly unpredictable weather as warm air masses, often from the Atlantic Ocean and the Gulf of Mexico, start moving north as temperatures rise. These warmer air masses clash with cold air masses lingering around from the winter and create big thunderstorms and tornadoes. The greater the temperature and moisture levels between these air masses, the more intense the weather.
Ch. 10: Extremely Summer
- Defining Summer — Technically, the first day of summer is June 21, although many places across the U.S. feel summer weather well before then. June 21 is the day of the ‘summer solstice’, when the northern hemisphere receives as much of the Sun’s rays as possible. This is the “longest day of the year.” The Sun is directly on top of us in the summer, resulting in hot temperatures across the U.S. From June 21 onward, the hours of daylight will become shorter as the Sun heads for the southern hemisphere. In the U.S. the further south you live, the earlier you will feel the summer weather and more intense you will feel the heat. This is partly because you are closer to the Equator (where it is always hot) than states in the northern half of the country.
- Dry Heat vs. Wet Heat — The summer heat in the western half of the U.S. is typically a “dry heat,” while the East and southeast experience a “wet heat” with a lot of humidity and rainfall. In the west, this has a lot to do with the Pacific Ocean, where the water is cooler than the Atlantic Ocean and Gulf of Mexico. As a result, the air above the Pacific Ocean is much drier than the air above the Atlantic Ocean and Gulf of Mexico, where the water is warm and evaporates quickly, throwing a lot of water vapor into the air. Because a lot of water vapor moves in from the Atlantic Ocean and Gulf of Mexico during the summer months, the Midwest and East Coast can experience quite a few very quick storms as the warm, moist air rises, cools to its dew point, and condenses into clouds that produce rain.
- Quote (P. 228): “At the water’s surface, evaporation is putting a lot more water vapor into the air above the Southeast’s warm Gulf Stream than the West Coast’s cold California Current.”
- The UV Index — Ultraviolet rays, which make up a large part of the sunshine we experience every day, can be dangerous. Many of these UV rays are filtered out by the ozone high up in the atmosphere, but not all of them. UV rays cause things like sunburn and skin cancer. During the summer, when we experience the most sunshine, levels of UV radiation can be hazardous. The National Weather Service operates a daily UV Index Forecast that predicts the intensity of UV radiation in a city. The scale ranges from 0-15.
- Quote (P. 231): “Scientists have isolated the dangerous part of the sunlight. It’s the ultraviolet rays that travel at a wavelength of light that you and I can’t see. A layer of ozone high in the atmosphere filters out a lot of the UV radiation, but not all of it, and during summer, when the Sun is highest in the sky, levels of UV radiation can be especially hazardous. The National Weather Service and other forecasting services have begun issuing a daily UV Index Forecast for many cities around the United States. Weather scientists measure the ozone layer and other features like cloudiness and predict the intensity of the ultraviolet radiation reaching within about 30 miles of the city. It ranges from 0 to 15, from low to high UV intensity.”
- Land = Big Reason for Heat — One of the main reasons many areas experience intense heat in the summer is due to the fact that land and ocean handle the Sun’s energy differently. The ocean has a higher heat capacity, meaning it absorbs and retains the Sun’s energy more slowly and gradually, leading to more moderate temperatures in nearby regions. Land, on the other hand, heats up much faster when exposed to the Sun, especially during the summer when we receive the most sunlight. This faster absorption of heat over land creates warm air masses above it, which contributes to the hot temperatures. Additionally, land cools down much more quickly overnight, leading to greater extremes in temperature in the winter, while the ocean’s slower heat absorption and release produces more stable, moderate temperatures in the areas next to it (i.e. the coast).
- Summer = Milder Weather — Generally, summer weather across the country is pretty tame compared to the chaos that can ensue in the winter and spring. This is largely because most of the country experiences warm weather. Because warm air masses cover most of the country, there are very few cold air masses hanging around. It’s the clash between cold, dry air masses and moist, warm air masses that cause such crazy weather, and these are exactly the conditions that are common in the winter and spring months. Overall, the differences in temperature and moisture levels between the air masses in the summer are far smaller than the winter and spring, resulting in generally less chaotic weather. However, water vapor moving in from the Atlantic Ocean and Gulf of Mexico during the summer months does cause quite a few quick storms in places like the East, Midwest, and Florida.
- Quote (P. 233): “Also, the warm temperatures of summer are more evenly spread around than the cold temperatures of winter. There is a bigger difference in the intensity of sunshine between north and south in the winter than the summer. The mid-latitude storms, which feed off the differences between warm and cold air masses, are strong in winter and weaker in summer. In January, most often there is a big difference between temperatures in Houston, Texas, for example, and Minneapolis, Minnesota. In summer, however, most of the time a big, warm blanket of air covers the entire region, and the temperature differences are not as great.”
- “Stable Air” — Previous chapter notes have discussed “unstable air,” a term used to describe upper atmosphere air that is colder than rising warm air. Because this air above is colder, the warm rising air can continue to climb higher and higher, causing more and more of its water vapor to condense and form clouds. These conditions can create big storms. In contrast, “stable air” is used to describe conditions where upper atmosphere air is warmer than the rising warm air. Because this air above is warmer, the warm rising air doesn’t climb as high. It gets suppressed closer to the surface and isn’t able to release into higher altitudes. This creates a situation where the warm air is “trapped” and temperatures get hotter and hotter. These conditions contribute to summer heatwaves, which can be deadly. And because the warm air can’t escape, it stays pretty warm overnight as well. Think about the warm nights in Arizona — the place simply can’t cool off overnight thanks to these “stable air” conditions that effectively put a lid over the region.
- Quote (P. 233): “If the air is warmer overhead than it is at the surface, the building heat will be prevented from escaping up into the higher altitudes. These conditions form a temperature inversion that acts as a lid over the region, preventing the normal process of nighttime cooling.”
- Chapter Takeaway — Across the country, the weather in the summer months is generally a lot less chaotic than the spring and summer. This is because cold air masses are fairly uncommon (the whole country is warm), leading to very few clashes between warm and cold air masses. And it is these clashes between warm and cold that can lead to intense weather in the winter and spring. Summer still produces quite a bit of rainfall in the Midwest, East, and Florida thanks to the water vapor moving in from the warm waters of the Atlantic Ocean and Gulf of Mexico, but the weather is generally less crazy across the board. The cold water in the Pacific Ocean causes those air masses out west to be dry rather than moist, leading to less rainfall.
Ch. 11: Falling for Autumn
- Defining Fall — Fall technically begins on September 22. This is the day that we are midway between the official first day of summer (June 21) and the first day of winter (December 21). Of all the seasons, fall is the tamest. Crazy things can still happen, but the weather is generally pretty good everywhere in the early part of fall. Like summer, this is largely because the air masses above are pretty similar in temperature and humidity (i.e. moisture) levels, which means fewer big clashes between warm and cold air masses.
- Interesting Fact — In the fall, leaves don’t exactly “turn” red, orange, or yellow — they “reveal” these colors. During the spring and summer, chlorophyll, which is green, is produced in large amounts to help trees and plants make food through photosynthesis. This green pigment covers up the red, orange, and yellow pigments that are present in the leaves all year long. As the days shorten in the fall and sunlight decreases, the chlorophyll breaks down and fades away, allowing us to see the underlying red, orange, and yellow pigments that are always there.
Ch. 12: Taking Care of the Air
- Stable Air & Pollution — In chapter 10, the concept of stable air was discussed. To recap, this is when the air in the upper atmosphere is warmer than the rising up into it from below. When this happens, warm air is unable to escape and gets “trapped” closer to the surface. In a temperature inversion like this, heat isn’t the only thing that is trapped below; the gases involved in pollution are also unable to escape into the upper atmosphere. When this happens, you can actually see the smog and pollution in the air. Think about the pollution and smog that are very visible some days in a big city like Phoenix, AZ, where emissions are being pumped into the atmosphere constantly. This is the result of stable air suppressing polluting gases.
- Quote (P. 256): “During a temperature inversion (e.g. stable air conditions), the layer of warmer air is like a lid, preventing the cooler air near the surface from rising and carrying away its polluting gases.”
- Pollution, Greenhouse Gases, and Global Warming — Stuff that pollutes the air comes in several varieties. Many of them are gases. Several of these gases are greenhouse gases, which trap heat like the roof of a greenhouse and prevent it from radiating out into space. These gases act very similar to stable air conditions mentioned in the bullet above. The global warming debate often involves discussion of these greenhouse gases (e.g. carbon dioxide, methane, and nitrous oxide). Many scientists believe that the buildup of greenhouse gases in our atmosphere is causing heat to be trapped closer to the surface, contributing to the rapidly rising average temperatures we continue to experience. We need greenhouse gases to sustain life on Earth, but human activities like burning fossil fuels and the emissions from driving cars are leading to a buildup of them in our atmosphere.
- Quote (P. 256): “Stuff that pollutes the air comes in several varieties. Many of them are gases. Several are what are called greenhouse gases, which trap heat like the roof of a greenhouse and prevent it from radiating out into space. These gases are what global warming is all about — concern by many of the world’s leading climate scientists that the greenhouse gases are building up to the point where they could be causing the atmosphere to warm up more than it would naturally.”
- The Hole in the Sky: The Ozone Layer — Chapter 10 briefly discussed ultraviolet (UV) rays from the Sun. These are the “killer rays of sunlight”; they cause sunburn, skin cancer, and eye damage. It turns out we also have the protection of the ozone layer in the atmosphere. This layer is made up of a rare form of oxygen. Without the ozone layer, we would all be dead — the Sun’s sunlight would kill us. The ozone layer filters out most of the Sun’s UV rays, but not all of them. Sunscreen helps protect us from the UV rays that get through. But the ozone layer is very thin, and the polluting gases we continue to pump into the air are eating away at the shield. In fact, it has reached a point where there is a well-known ozone hole in the sky over parts of the world like Antarctica. The hole is recovering thanks to a few key measures, but scientists are concerned that the ozone layer across the planet will continue to dissipate if we don’t stop pumping out so many emissions.
- Quote (P. 261): “The killer rays of sunlight are known as ultraviolet radiation, because the lengths of their waves are in the invisible range out beyond the color violet that you see. Some of this radiation — called UV-B — causes sunburn, skin cancer, and eye damage.”
- Quote (P. 261): “The only thing between you and these deadly rays [UV rays] is a thin layer of gas high in the sky above where the weather takes place. It’s between 9 and 30 miles up, in the band of sky known as the stratosphere. The gas is a rare form of oxygen known as ozone.”
- Quote (P. 261): “The bad news is, because of air pollution, this thin shield of ozone is getting even thinner. Some chemical reactions are taking place up there in the stratosphere that are eating the ozone. It has reached the point to where an ozone hole forms in the sky over some parts of the world.”
- Quote (P. 262): “The only equation you need to keep in mind is this: Less equals more. Less ozone in the stratosphere over your head means more lethal ultraviolet radiation in your face.”
- CFCs & The Ozone — For many years in the mid-1900s, we relied on products called CFCs (for chlorine, fluorine, and carbon) for a wide range of things, including to help operate our refrigerators and air conditioners. The problem is that these CFC gases are some of the worst possible gases for the ozone. They eat away at the ozone layer and can float around up there for 50-100 years. Once scientists proved how bad these products were for the environment, they were eventually banned in the 1980s and 90s.
- On Climate Change & Global Warming — Climate change is natural. Over the course of its 4.5 billion years, Earth has been both significantly warmer and cooler than it is today. In the last century, the steadily increasing average global temperature has given rise to the hotly-contested “global warming” debate. In short, the average temperature of Earth has warmed one degree in the last century, and scientists expect another 2-6 degrees in the next century. It doesn’t seem so, but that’s significant. And it’s a far faster pace than ever. The global warming debate mostly centers around whether or not this warming is natural or unnatural. Is it part of the Earth’s natural ebbs and flows in climate, or are the greenhouse gases we continue to pump into the air the cause? We have always had greenhouse gases in our atmosphere — they trap heat radiating up from the surface (remember, much of the Sun’s energy goes through our atmosphere and rebounds back up from the surface) and keep us warm. Without them, we’d be dead. Water vapor and carbon dioxide (CO2) are the two main greenhouse gases. The problem is that, since the Industrial Revolution, human activity has pumped a huge amount of CO2 and other greenhouse gases into the air via the burning of fossil fuels, burning forests, and car emissions. Through these activities, scientists agree that we now have too many greenhouse gases in the atmosphere, and those gases are contributing to the rapid warming of our planet by trapping heat closer to the surface.
- Quote (P. 267): “In a way, global warming is a case of too much of a good thing. Just as climate shifts are natural, so is the ‘greenhouse effect’ that people are talking about when they describe global warming. If it were not for the greenhouse effect — the fact that gases in the atmosphere trap heat radiating up from the surface — Earth would be too cold for living things.”
- Quote (P. 267): “Here’s what scientists mean by global warming: It’s the heating up of the atmosphere on top of the natural greenhouse effect, which is caused by the gases that humans have added to the atmosphere since the Industrial Revolution began a couple hundred years ago. It’s the unnatural rise — and unnaturally rapid rise — in temperatures caused by the fact that you and I have changed the chemical makeup of the atmosphere.”
- Quote (P. 268): “Not much about this global warming business is simple, except maybe its cause: More than anything, it’s the gases that are byproducts of the burning of what scientists call fossil fuels — coal and oil and natural gas that come from the decay of old plants and animals. They are carbon dioxide, nitrous oxide, and methane. All these are naturally occurring ingredients of the atmosphere, but human activities have raised their proportion and changed the mix.”
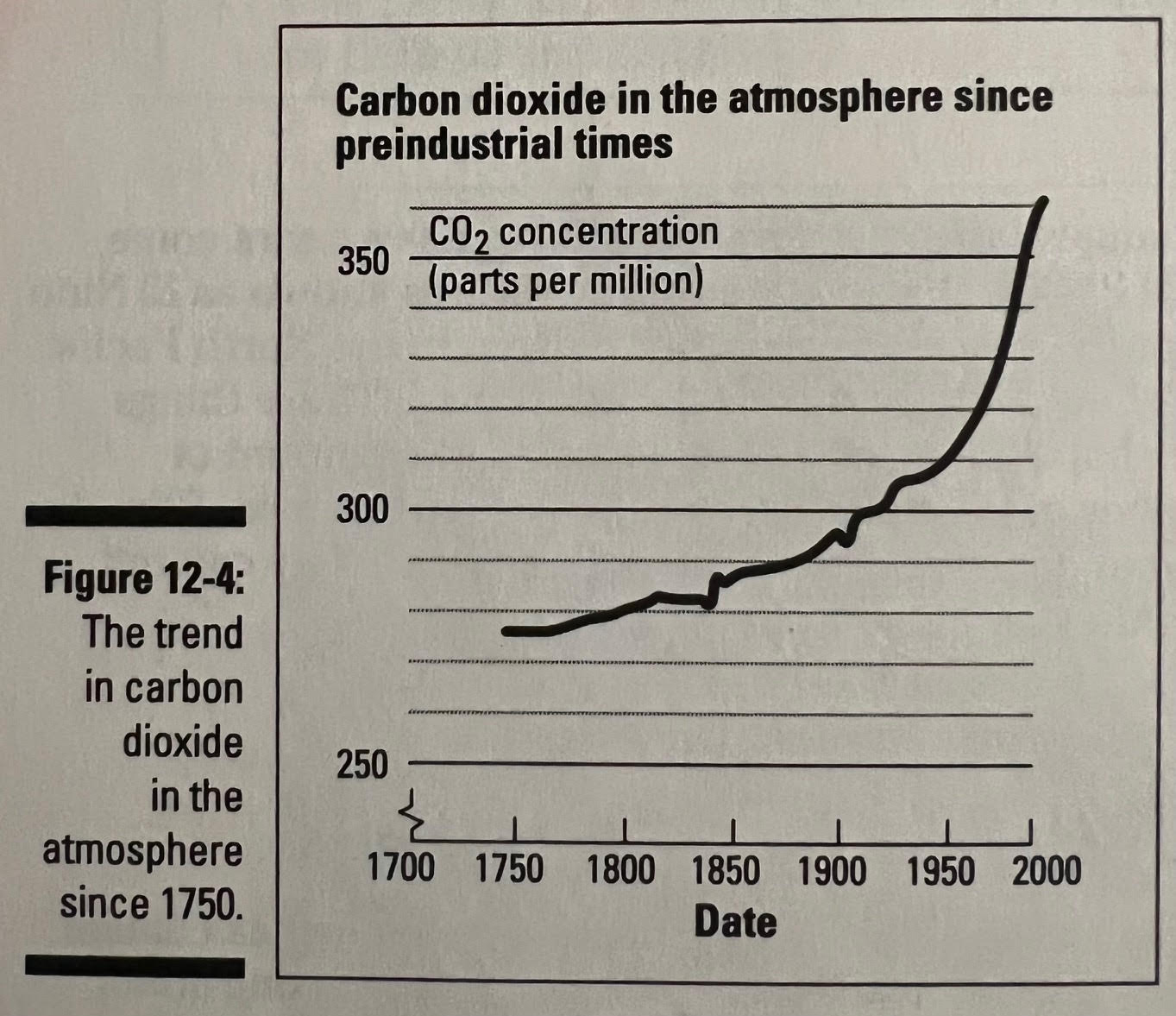
- Chapter Takeaway — The global warming debate centers around how human activity is contributing to the buildup of greenhouse gases in our atmosphere. These gases (e.g. carbon dioxide, methane, and nitrous oxide) have always been in our atmosphere and help keep the planet warm by trapping heat close to the surface. Without them, Earth would be way too cold to live on. But human activity has put a huge amount of additional greenhouse gases into our atmosphere — particularly CO2. Scientists believe this human activity and buildup of greenhouse gases is causing the rapid climb in average global temperature that we call “global warming.”
Ch. 13: Up in the Sky! Look!
- Colored Skies: Why Is the Sky Blue? — All of the colors we see in the sky during the daytime, even the plain blue sky, are the result of the atmosphere scattering the Sun’s light. The Sun’s light is made up of these colors: red, orange, yellow, green, blue, indigo, and violet. Red and orange are the longest of the light waves, while blue, indigo, and violet are the shortest. When all of these light waves strike equally on the rods in our eyes, we see white sunlight. But when we look up and see blue sky, or a red and orange sunset, these colors are the result of stuff in the atmosphere (e.g. air molecules, rain drops and ice crystal in clouds, polluting gases) scattering the light waves, making only certain colors visible to our eyes. For example, the tiny nitrogen and oxygen molecules that make up air selectively scatter the shortest wavelengths (blue) and allow the long ones (red and orange) to pass through. This is why we see the sky as blue; the air molecules are scattering the short blue rays in all directions. In short, the stuff in the air is interacting with sunlight to create the colors we see in the sky. At night, without sunlight to scatter and reflect, there is no light to interact with the atmosphere, resulting in pitch black skies.
- Quote (P. 274): “The light of day is a mixture of different waves of energy radiating from the Sun. Each wave has a different length, as the next section describes, and each length is seen as a different color. When all the different waves strike about equally on the rods and cones in the back of your eye, you see white light.”
- Quote (P. 275): “The scattering and the bending of the sunlight and the other processes that separate the wavelengths into the various colors are happening all the time. The bright white light of the Sun over your head at high noon on a summer day is at that very moment somebody else’s red and orange sunset. It’s a matter of angle or slant. It’s also a matter of the stuff in the sky between the sun and the color-seeing rods and cones in the backs of your eyeballs.”
- Quote (P. 274): “The sky is always scattering light waves. The tiny gas molecules are scattering short waves, making the sky blue. The clouds are scattering all wavelengths, which is what makes them white. Sunsets are red, orange, and yellow because these colors are scattered near the ground as the sunlight passes through the atmosphere at a sharp angle.”
- Quote (P. 275): “The sky is blue because when light from the Sun comes down to Earth, it bounces off the tiniest parts of the air, and when this happens, the tiny parts of the light that make the color blue scatter out everywhere. Because they are so small, the molecules of the gases oxygen and nitrogen, which make up most of the air, selectively scatter only the shortest wave length rays of sunlight and allow the longer wavelength rays to pass on through. When you look up on a clear day, you are seeing those short wave length blues that the air molecules are scattering in all directions.”
- Why Are Clouds White? — Building on the point above, clouds are white because the water drops and ice crystals within them are scattering all of the light waves that make up the Sun’s sunlight evenly. When all of the colors of sunlight are scattered in equal proportions like this, we see white light. Again, all of the little nitrogen and oxygen molecules in the air are scattering the short blue rays much more than the long red and orange, which is why the sky is blue. All of the colors we see in the sky are the result of this STUFF in the air (e.g. air molecules, rain drops and ice crystals in clouds, polluting gases) scattering different colors of sunlight in different proportions.
- What Causes Sunrises & Sunsets? — If the ideas in the first two bullets are true, why do we see beautiful red and orange skies when the Sun rises and sets? In the case of sunrises and sunsets, the Sun is located lower in the sky than it is in the middle of the afternoon, when it is high up in the sky overhead. When the Sun is lower in the sky like this, the light waves that comprise sunlight must travel further through the atmosphere, causing the waves to strike air molecules and clouds in the sky at a greater angle. This increase in distance and angle that the light waves must travel causes more of the light to both bend and scatter before it hits our eyes. Eventually, as the Sun gets lower and lower during a sunset, the light waves have to travel so far to reach our eyes that only the long waves of red and orange are left. This is a sunset. And it happens as the Sun rises during a sunrise as well. This is not the case when the Sun is way up in the sky overhead midday; in that high in the sky position, all of the Sun’s light waves are traveling a shorter distance to reach us and are striking air molecules at a steady angle. The allows the shorter blue rays to dominate, causing blue skies.
- Quote (P. 279): “On a clear summer day, when the Sun is high in the sky, all wavelengths of its light are arriving at your eyes with about the same intensity. The sunshine is brightest and whitest about noon. The Sun had risen in the east in a red and golden glow, and all morning long as it climbed the sky, it faded from orange to yellow to the bright white of noon. These changes in color are caused by the different amounts of atmosphere that the rays of sunlight must travel through at different times of day on their way to your eyes. As its arc shifts lower in the sky through the afternoon, the rays of light strike through the atmosphere at a greater and greater angle. The process causes more and more of the light to both bend and scatter. The lower the Sun sinks toward the horizon, the more atmosphere its rays must travel through, and the more scattering of the short wavelengths of light by the air molecules. Finally, as the Sun nears the horizon, its rays travel through so much atmosphere on their way to your eye that all that is left is the long wavelengths — the yellows and oranges and reds of sunset.”
- How Do Rainbows Form? — When you see a rainbow, you are looking at falling rain that is reflecting sunlight. A rainbow happens when sunlight shines through raindrops in the sky. For a rainbow to appear, the Sun must be shining and behind you, and the rain is in front of you. When sunlight enters a raindrop, its light waves (red, orange, yellow, green, blue, indigo, and violet) bend and reflect, like bouncing off a mirror. The light splits into different colors because the light waves bend at different angles. Colors like red, orange, and yellow bend less, while blue, green, and violet bend more. These colors spread out and create the rainbow we see. Each person sees their own rainbow because the light enters their eyes from different raindrops. Rainbows can also appear in mist from sprinklers or waterfalls when the Sun shines through them. Rainbows are really all about the Sun shining light on falling rain drops. Typically we don’t see rainbows when it’s raining because the Sun isn’t out. In the rare occasion that the Sun is out and shining in part of the sky while rain falls in another area, you can sometimes see a rainbow.
- Quote (P. 282): “Two interesting things happen to the sunlight that has passed over your head when it reaches the drops of falling rain. The sunlight is bent, or refracted, and also it is bounced back, or reflected. The white sunlight enters the raindrop, reflects off the back side of the drop of water as if it were a mirror, and then goes back out the same side it entered.”
- Quote (P. 282): “Rainbows occur only when it is raining in one part of the sky and the Sun is shining in another.”
- Quote (P. 327): “The effect of a rainbow depends on sunlight reflecting off of falling raindrops and bouncing back toward your eyes. The Sun needs to be at your back.”
- Chapter Takeaway — Together, light from the Sun and the gases that make up our atmosphere can create quite a show in the sky. The blue sky of a clear day, the vibrant red and orange colors of a sunrise or sunset, and spectacles like rainbows are all the result of light waves (e.g. red, orange, yellow, green, blue, indigo, and violet), from the Sun being scattered, bent, and reflected by the stuff in the atmosphere, such as air molecules (nitrogen and oxygen), rain drops and ice crystals in clouds, and even pollution. Without this stuff in the atmosphere to scatter and bend the light, we would live in a world of plain, bright white light. It is the scattering and bending of the Sun’s white light that gives our world color.