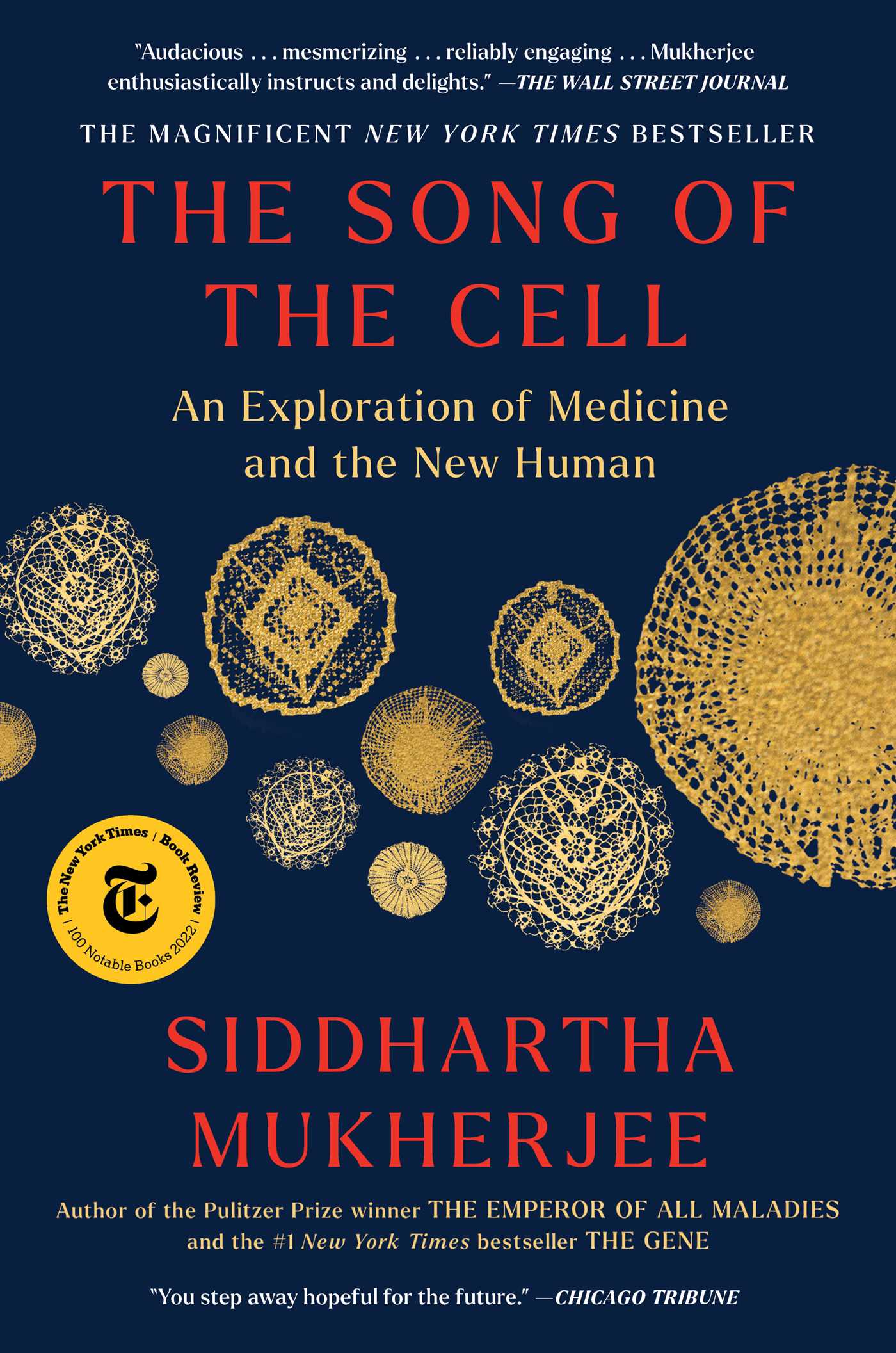
The Song of the Cell
Siddhartha Mukherjee
GENRE: Health & Wellness
PAGES: 496
COMPLETED: February 28, 2024
RATING: 




Short Summary
Cells are the building blocks of the body. The units of life. But what exactly do they do? How do they work together to keep the body functioning, and what happens when they begin to misbehave? In The Song of the Cell, renowned author and cancer physician Siddhartha Mukherjee tackles these questions, reflecting on the history of cell biology and how modern scientists and physicians are reengineering cells to help patients dodge diseases, cure cancer, and live healthier lives.
Key Takeaways
Cells: The Units of Life — We are made of 37 trillion individual cells; 37 trillion tiny labs that carry our genetics, perform specific work, and have their own special functions. But all of us start as one single cell. That one cell divides furiously until our tissues, organs, and body are fully constructed. In this way, we are the sum of parts. Life is, in general, cell activity. All living organisms — plants, animals, humans — are made of cells.
Dysfunctional Cells = Dysfunctional Body — All diseases and illnesses of the body and mind (e.g. cancer, heart failure, diabetes, hip fracture, etc.) are the result of cells in the body not functioning correctly. Disease and illness can be traced back to cellular dysfunction; when certain cells are behaving abnormally, it manifests as disease in the body. When cells are operating normally, the body is well. Cancer is maybe the most frightening case of cellular dysfunction. Cancer cells often go undetected by our immune system, don’t follow the normal rules of cell division and growth, and move to areas of the body they shouldn’t be. Their goal is to divide until they overtake the body.
Building ‘New Humans’ — Through cellular engineering, we are redefining medicine and the human experience. Scientists and researchers are devising new ways of editing cells, and the genes they carry, to augment and enhance our lives. The potential is huge. For instance, we’ve already developed an effective immunotherapy for leukemia that involves drawing out T cells, beefing them up in a lab, and reinserting them back into the body to fight cancerous cells. Sickle cell disease is being cured by editing the genes in hemoglobin, a protein located in each of our red blood cells that carries oxygen. Cell and gene editing is the future of medicine.
Favorite Quote
“The discovery of cells, and the reframing of the human body as a cellular ecosystem, also announced the birth of a new kind of medicine based on the therapeutic manipulations of cells. A hip fracture, cardiac arrest, immunodeficiency, Alzheimer's dementia, AIDS, pneumonia, lung cancer, kidney failure, arthritis — all could be reconceived as the results of cells, or systems of cells, functioning abnormally.”
Book Notes 
Prelude
- About the Author — Siddhartha Mukherjee is the author of this book. He is an associate professor of medicine at Columbia University and a cancer physician and researcher. A Rhodes scholar, he graduated from Stanford University, University of Oxford, and Harvard Medical School. He is the author of The Gene: An Intimate History, a #1 New York Times bestseller; The Emperor of All Maladies: A Biography of Cancer, winner of the 2011 Pulitzer Prize in general nonfiction; and The Laws of Medicine.
- Cells: A Life Within a Life — In 1837, Matthias Schleiden and Theodor Schwann discovered that cells were the building blocks in both plants and animals. The two were having dinner in Berlin and discussed the presence of cell-like objects in the subjects they were studying; as a botanist, Schleiden was working with plants and Schwann, a zoologist, was working with animals. Although they weren’t the first to discover cells, they were the first to recognize that cells were fundamental units of all living organisms. Cells are “a life within a life.” Every cell is an independent living being — a unit — that forms a part of a whole.
- Quote (P. xvi): “[Cells are] a life within a life. An independent living being — a unit — that forms a part of the whole. A living building block contained within the larger living being.”
- Quote (P. xvi): “That evening in Berlin, he would later recall, the two friends [Schleiden and Schwann] had converged on a universal and essential scientific truth: both animals and plants had a ‘common means of formation through cells.’”
- Quote (P. xvii): “Schleiden and Schwann weren’t the first to see cells, or to realize that cells were the fundamental units of living organisms. The acuity of their insight was in the proposition that a deep unity of organization and function ran through living beings.”
- About the Book — Mukherjee’s goal with this book is to tell the story of the cell: where it was discovered, how it works, and how we are now doing some interesting things to cells today in an effort to solve medical problems. It’s a story of how clusters of living cells create tissues, organs, and organ systems, and how cells enable immunity, reproduction, cognition, repair, and more. It’s also the story of what happens when cells become dysfunctional, tipping our bodies from cellular physiology into cellular pathology — the malfunctioning of cells that lead to problems in our body. Finally, it’s about how our deepening understanding of cellular physiology and pathology has sparked a revolution in biology and medicine, leading to the birth of transformational medicines.
Introduction
- Cancer Cells — Cancer develops when cells become destructive and begin attacking the body. One of the reasons cancer develops into a deadly disease is that these destructive cancer cells go undetected by the body’s T cells, which are basically assassins in the immune system whose job is to kill off bad, infected, faulty cells. T cells are what help hunt and kill virus-infected cells when we have the flu. In other words, cancer cells go about their business undetected by T cells.
- Immunotherapy — An immunotherapy is any therapy that tries to boost or harness a person’s immune system to fight an infection or other condition (example: vaccines). Immunotherapy is a type of cell therapy. One type of immunotherapy that doctors and scientist are working on involves extracting T cells from the body of a cancer patient, reengineering them and arming them with “weapons” in lab, then inserting them back into the body so they can detect and eliminate cancer cells that were previously undetectable.
- Quote (P. 5): “Emily’s trial involved infusing her body with her own T cells. But these T cells had to be weaponized, via gene therapy, to recognize and kill her cancer.”
- Cells & Health Issues — A cell is a unit of life. We are made of a ton of cells (37 trillion), therefore most, if not all, diseases and health issues in the body are a result of cells not working correctly. Hip fractures, heart attacks, AIDS, dementia — every issue involves cells acting abnormally. Immunotherapies like the one described above are part of a new and promising wave of medicine that often involves manipulating and reengineering cells to be better and stronger.
- Quote (P. 7): “As I watched the advance and retreat of Sam’s illness, and the remarkable recovery of Emily Whitehead, I knew that I was also observing the birth of a kind of medicine in which cells were being repurposed as tools to fight illness-cellular engineering. . . We are built out of cellular units. Our vulnerabilities are built out of the vulnerabilities of cells. Our capacity to engineer or manipulate cells (immune cells, in both Sam’s and Emily’s cases) has become the basis of a new kind of medicine — albeit a kind of medicine that is still in midbirth.”
- Quote (P. 9): “The discovery of cells, and the reframing of the human body as a cellular ecosystem, also announced the birth of a new kind of medicine based on the therapeutic manipulations of cells. A hip fracture, cardiac arrest, immunodeficiency, Alzheimer’s dementia, AIDS, pneumonia, lung cancer, kidney failure, arthritis — all could be reconceived as the results of cells, or systems of cells, functioning abnormally.”
- New Medicine: Reengineering Cells — Modern science is very focused on “cell therapy,” which is basically the act of modifying and reengineer cells to alleviate health issues. There are four main ways we are going about the process of changing and modifying cells via cell therapy:
- Use of Drugs — The first is the use of drugs, chemical substances, or physical stimulation to alter the properties of cells — their interactions with one another, their intercommunication, and their behavior. Antibiotics against germs, as well as chemotherapy and immunotherapy for cancer, fall in this first category.
- Transfer of Cells — The second is the transfer of cells from body to body (including back into our own bodies), exemplified by operations like blood transfusions and bone marrow transplantations
- Synthesis — The third is the use of cells to synthesize a substance (insulin or antibodies) that produces a therapeutic effect on an illness
- Genetic Modification — This involves modifying the actual genes of cells to create cells, organs, and bodies that have new properties and abilities. This is the act of literally reengineering cells, much like the T cell immunotherapy described a few bullets above.
- The New Human — As advances are made in this new form of medicine where cells are modified, rebuilt, and reengineered to combat health problems and suffering, we are giving rise to what Mukherjee calls “the new human.” A few examples of how this type of medicine is being developed to create “new humans” include:
- Diabetes — A type 1 diabetic infused with his own stem cells that have been engineered to produce the hormone insulin to maintain a normal blood glucose level
- Heart Issues — An octogenarian who, following multiple heart attacks, is injected with a virus that will home to his liver and permanently lower artery-clogging cholesterol, thus reducing his risk of another cardiac event
- Depression — A woman with crippling, recalcitrant depression whose nerve cells (neurons) are being stimulated with electrodes.
- What Is a Cell?: A Gene Decoding Machine — Cells do a lot of things, but one of the most important functions they carry out involves decoding genes. Put simply, they are a decoding machine for genes. Genes provide instructions (code) to build proteins, which perform almost all work in a cell. Proteins enable biological reactions, coordinate signals within the cell, and turn genes on and off to regulate a cell’s identity. Genes are physically located in a molecule called DNA, which is packaged inside every single one of our cells as chromosomes. here’s the big points: Genes need cells; without them, the code (instructions) they carry for proteins would not be read or acted on in the body. Without cells, our genes would be lifeless.
- Quote (P. 11): “By decoding, I mean that molecules within a cell read certain sections of the genetic code, like musicians in an orchestra reading their parts of a musical score — the cell’s individual song — thereby enabling a gene’s instructions to become physically manifest in the actual protein. Or, put more simply, a gene carries the code; a cell deciphers that code. A cell thus transforms information into form; genetic code into proteins.”
- Quote (P. 12): “A gene without a cell is lifeless — an instruction manual stored inside an inert molecule, a musical score without a musician, a lonely library with no one to read the books within it. A cell brings materiality and physicality to a set of genes. A cell enlivens genes.”
- What Is a Cell?: An Integrating Machine — By decoding a gene’s instructions, thereby activating the protein molecules in the cell, the cell becomes an integrating machine. The cell basically uses its set of proteins to start coordinating the common behaviors of a cell, things such as movement, metabolism, signaling, delivering nutrients to other cells, and surveying for foreign objects. And those behaviors in turn manifest as the behavior of the organism (e.g. humans, plants, animals, etc.). Therefore, if cells are functioning correctly, the organism (us) functions correctly. Any diseases or health issues that come up for us are a result of cells not functioning correctly.
- Quote (P. 12): “The metabolism of an organism reposes in the metabolism of the cell. The reproduction of an organism reposes in the reproduction of a cell. The repair, survival, and death of an organism repose in the repair, survival, and death of cells. The behavior of an organ, or an organism, reposes in the behavior of a cell. The life of an organism reposes in the life of a cell.”
- What Is a Cell?: A Dividing Machine — Finally, cells are dividing machines. Molecules within a cell (again, these are proteins) initiate the process of duplicating the genome. The internal organization of the cell changes. Chromosomes, where genes are physically located in the cell, divide. Cell division is what drives the fundamental features of life, such as growth, repair, regeneration, and reproduction.
- Cells & COVID-19 — Dysfunctional cells were at the heart of the COVID-19 pandemic. Just like all diseases and health issues we encounter, COVID was a result of cells not functioning properly. A virus is a problem, but it’s nothing without cells. In the case of COVID, our immune cells were “misfiring,” causing a lot of respiratory issues for people who had the virus.
- Quote (P. 14): “The Yale University virologist Akiko Iwasaki told me that the central pathology caused by SARS-CoV2 (severe acute respiratory syndrome coronavirus 2) was ‘immunological misfiring’ — a dys-regulation of immune cells. I had not even heard the term before, but its immensity hit me: at its core, the pandemic, too, was a disease of cells. Yes, there was the virus, but viruses are inert, lifeless, without cells. Our cells had awoken the plague and brought it to life.”
- Chapter Takeaway — Cells are units of life present in every organism. They are living, breathing things that decode genes, allowing protein molecules within the cell to perform their duties. Any disease or health issue we encounter (e.g. cancer, heart attack, hip fracture) is ultimately the result of certain cells in our body not functioning correctly. Modern science is very focused on cell therapies, which involve modifying and reengineering cells in an effort to alleviate health issues and suffering.
Ch. 1: The Original Cell
- First, There Was One — The human body is made up of about 37 trillion cells, but we all started as one single cell (a single-celled embryo). The first cell gave rise to a few more cells, which then divided into even more cells. Our cells continued to divide furiously until our liver and guts and brains, and every other piece of our body, was formed.
- Anatomy vs. Pathology — Anatomy is the study of structural elements of the human body; for example, what the kidneys are and where they are located in the body. Pathology is the study of human diseases and their causes.
- Early Confusion About Disease — In the 16th and 17th centuries, there was massive confusion about what causes disease. Although people knew of cells, nobody knew a lot about them or how they functioned. At the time, most diseases were thought to be from “miasmas,” poisonous vapors coming from sewage or contaminated air. The miasmas, so it was thought, carried particles of decaying matter called “miasmata” that somehow entered the body and caused decay. In short, disease was thought to be a condition of uncleanliness or contamination. While there is some logic there, this theory was obviously wrong. Disease occurs when cells aren’t functioning correctly.
- Chapter Takeaway — Prior to big advances in cell biology in the late 1700s and 1800s, nobody really knew what caused diseases. Although people knew of cells, nobody knew a lot about them or how they functioned. The commonly held belief was that diseases were caused by poisonous vapors coming from places like the sewage system.
Ch. 2: The Visible Cell
- Inventing the Microscope — The microscope as we know it today was born in the early 16th century, when Hans and Zacharias Janssen, a Dutch father-son team of opticians, fixed two magnifying lenses on the top and bottom of a tube. They found a way to rig the device in a way that allowed a user to magnify things and witness their properties up close.
- Witnessing Cells — In 1675, Antonie van Leeuwenhoek built his own microscope, following the Janssen model, and saw cells in action after gathering some rain water and putting a few drops under his scope. The drops of water were filled with thousands of tiny organisms that darted around like crazy. He called these organisms “animalcules” at the time, but what he had witnessed was living cells. While bored during COVID, Mukherjee, the author of this book, wanted to see this for himself and decided to recreate the moment. Below is a vivid account of what he saw:
- Quote (P. 29): “The drop came sharply into view, and then a whole world within it. An amoeboid form flashed across the lens. There were branches of an organism I could not name. Then a spiral organism. A round, moving blob, surrounded by a halo of the most beautiful, the most tender filaments that I had ever seen. I could not stop seeing. Cells.”
- The Cell Is Discovered — A decade before Antonie van Leeuwenhoek witnessed cells in rain drops, Robert Hooke, an English scientist, had also seen cells, although not live ones. He observed the outer walls of dead cells in 1965 when looking at a thin slice of cork that he had sliced off of a plant stem. He decided to call them cells, from “cella,” a Latin word meaning “small room.” That’s essentially what cells are — small compartments or little boxes that move and swim around freely. Hooke is credited with discovering the cell.
- Chapter Takeaway — The first legit microscope was invented by Hans and Zacharias Janssen in the early 1600s. The birth of the microscope eventually led to the first few magnified observations of cells, by Antoine van Leeuwenhoek and Robert Hooke in the 1650s and 1660s.
Ch. 3: The Universal Cell
- Francois-Vincent Raspail — Raspail was a French botanist, chemist, and microscopist who helped advance cell biology significantly in the 1800s. Building on the findings of Hooke and Leeuwenhoek, Raspail set out to learn what cells did, what they were made of, and where they originated. An early pioneer in the field, he wanted to advance cell biology past the point of merely acknowledging that cells existed. A few questions he set out to answer:
- What Are Cells Made Of? — Raspail determined that cells select from their surrounding environment and take only what they need. They are often made of elements like water and carbon.
- What Do Cells Do? — We now know exactly what cells do (see earlier notes from prelude and introduction), but at the time, Raspail theorized that every cell was its own little lab that performs chemical processes to make tissues and organs function. This theory was correct, although Raspail couldn’t necessary prove it because the technology back then wasn’t strong enough.
- Where Do Cells Come From? — Raspail posited that “from cells come cells.” In other words, he believed cells divided and created more cells, which was correct. All of his findings helped take cell biology to the next level.
- Cells as “Laboratories” — Raspail’s concept of the cell as a laboratory was groundbreaking. We are made up of billions of units of these little individual labs, each performing their own unique functions (e.g. muscle cells, sperm cells, red blood cells, white blood cells, etc.). As Mukherjee put it:
- Quote (P. 40): “The T cells that I had seen under the microscope in the Oxford lab were ‘Surveillance Labs,’ swimming in fluid to find viral pathogens hiding within other cells. The sperm cells that Leeuwenhoek had seen under his scope were ‘Information labs,’ collecting hereditary information from a male, packaging it in DNA, and attaching a powerful swimming motor to deliver it to the egg cell for reproduction.”
- Cell Theory: First Two Laws — As mentioned in the prelude chapter, Matthias Schleiden and Theodor Schwann discussed the presence of cells in plants and animals one night in Berlin in 1837. Their conversation eventually led to the first two principles of cell theory. Neither Schwann nor Schleiden had discovered anything new about cells; their big contribution was in collecting the work of Hooke, Leeuwenhoek, and other earlier pioneers of cell biology, and using it to propose that cells were not just present in certain animals or plants — they are present in every living thing. Cells made up all tissues in every living thing. They proposed a universal principle of biology that was rooted in the idea that cells build organisms. Their first two principles of cell theory were:
- All living organisms are composed of one or more cells
- The cell is the basic unit of structure and organization in organisms
- Cells Make More Cells — In the mid-1800s, there was still debate about where human cells came from. The “vitalists” of the time proposed that cells came from (or were made from) special chemicals or “vital fluid” exclusive to humans. In the 1850s, Rudolf Virchow put an end to the debate by adding a third principle to cell theory: Cells come from cells. Cells divide. This was confirming what Raspail had already theorized years earlier.
- Quote (P. 48): “But where did cells come from? As with Schwann and Schleiden, it was time for unifying maxims, and Virchow was ready. Every piece of evidence had been laid out by his predecessors; he merely had to pick up the crown and place it on his head. This feature of cells arising from cells wasn’t true just for some cells and some tissues, Virchow stated, but for all cells. It was not an anomaly or an idiosyncrasy, but a universal property of life in plants, animals, and humans. The division of one cell gave rise to two, and two to four, and so forth.”
- Quote (P. 49): “There was no need to invoke special chemicals or divine processes to describe the origin of a cell (as the vitalists had claimed). A new cell came from the division of a prior cell; that’s all there was to it.”
- Cell Division & Cancer — Virchow arrived at his conclusion about cell division after observing cancer patients. In one instance, the blood of a patient who had died of leukemia was filled with a huge number of white blood cells. His feeling was that cancer develops into a disease when cancer cells divide and spread like crazy. At the time, cell division wasn’t something that anybody really accepted, which is why some diseases like cancer were a complete mystery. Raspail had theorized about cell division years earlier, and a few other microscopists had witnessed it under a microscope, but the idea wasn’t a commonly accepted one at the time.
- Quote (P. 48): “Sickness or neglect in just one part could become a diffuse sickness of the whole, just as a single cancer cell could generate billions of malignant cells and precipitate a complex, deadly disease. ‘The body is a cell state in which every cell is a citizen,’ Virchow would write. ‘Disease is merely the conflict of the citizens of the state brought about by the action of external forces.’”
- Virchow & Cellular Pathology (Disease) — Virchow was the first person to connect disease in the body with dysfunction in cells. Pathology (disease in the body) is the result of cellular pathology (disease). This finding completely changed the world of medicine. Prior, the belief was that diseases were caused by the breakdown of tissues, organs, and organ systems. Virchow helped show that a failing heart, for example, is the consequence of failing heart cells. Cancer is the result of dysfunctional cells that are dividing and spreading.
- Quote (P. 50): “The words ‘function’ and its opposite, ‘dysfunction’, were crucial: normal cells did normal things to ensure the sanctity and physiology of the body. They were not just passive, structural features. They were actors, players, doers, workers, builders, creators — the central functionaries in physiology. And when these functions were somehow disrupted, the body fell into disease.”
- Quote (P. 55): “It isn’t sufficient to locate a disease in an organ; it’s necessary to understand which cells of the organ are responsible. An immune dysfunction might arise from a B cell problem, a T cell malfunction, or a glitch in any of the dozens of cell types that comprise the immune system. . . To locate the heart of normal physiology, or of illness, one must look, first, at cells.”
- Cell Theory: All Five Laws — Cell theory consists of five principles, the first two coming from Schwann and Schleiden, and the next three coming from Virchow. The three laws added by Virchow completely changed the world of medicine. These five principles formed the pillars of cellular biology and cellular medicine that we know today. They changed our understanding of the human body. An updated look at the five laws:
- All living organisms are composed of one or more cells
- The cell is the basic unit of structure and organization in organisms
- All cells come from other cells (cells divide)
- Normal physiology is the function of cellular physiology (i.e. the body and its organs and tissues function correctly when cells are functioning correctly)
- Disease in the body is a result of faulty cells that are not functioning correctly
- Chapter Takeaway — All of us start as one cell. That cell divides furiously until our organs, tissues, and body are complete. Disease and illness can be traced back to cellular dysfunction; when certain cells are not functioning correctly, it manifests as disease in the body. When cells are functioning well, the body is well. Rudolf Virchow was a major contributor to all of these findings and helped advance cellular biology in the 1800s.
Ch. 4: The Pathological Cell
- Microbes & Germ Theory — Microbes are actual living organisms that have just one cell. Although most microbes are completely harmless, some have the ability to invade human tissues and initiate inflammation, putrefaction (decaying/rotting), and deadly diseases. These facts make up germ theory, which states that microbes are independent, living cells capable of breaking down tissues and causing human illness. Prior to germ theory, people were unaware that microbes could cause disease; the belief was that bad air was caused diseases.
- Microbes & Pathogens — The effects on the human body that microbial cells (microbes) exhibited was one of the first clear signs to many scientists in the 1800s that cells are involved when the body is in a disease state. In other words, a microbial cell can cause an illness in the body, which made people realize that cellular dysfunction could be a big part of the story for any disease or illness of the body.
- Microbes vs. Viruses — Microbes are a collection of bacteria, fungi, etc. that live on and inside of you. Because they have a cell, a microbe is a living thing. They move, eat, and breathe. A virus doesn’t have cells and therefore is not a living thing. However, when a virus is breathed in or brought into the body somehow, therefore getting in touch with a human cell, it activates and becomes a living thing. And a real problem. Otherwise, though, a virus is a harmless thing because it’s not even alive.
- The Interesting Case of Ignaz Semmelweis — Ignaz Semmelweis was a Hungarian obstetrician who found that the reason two hospitals in his town exhibited far different mortality rates among women enduring childbirth had much to do with doctors not washing their hands properly. When he urged surgeons in the high death rate hospital to wash their hands with water and chlorine before operating, thus removing harmful microbes, the mortality rate dropped by 90%. Unfortunately, when he tried to spread the word to other hospitals, he lost his job and was shunned by doctors, most of whom didn’t believe in germ theory. He later suffered a mental breakdown and passed away.
- How Germs Work: Interruptors — External bad microbes/bacteria (which are single cell organisms) and germs can enter the body and cause disruption in physiology. This disruption can get worse if the single-cell microbes reproduce and multiply. All of this can lead to disease and illness. As an example, the bacterium vibrio cholerae is what causes cholera. In this way, germs and microbes/bacteria are interruptors; they invade your body and interrupt your physiology. Cancer, on the other hand, occurs when your human cells turn dysfunctional, divide, and rebel against you.
- Poor Hospital Hygiene — In the 17 and 1800s, poor hospital hygiene was the norm, causing crazy high death rates. Equipment was used without being sterilized and doctors would just use the same tool on every patient without washing it. They also wouldn’t wash their hands. As a result, patients were routinely exposed to bad bacteria and disease. Today, strict rules and guidelines at hospitals and health care offices prevent this kind of thing from happening.
- Antibiotics — Antibiotics, medicine designed to kill microbial cells, changed the course of medicine and were first introduced in 1910 to eliminate syphilis microbes. Soon, there was a wide variety of antibiotics, including penicillin, an antibacterial chemical secreted by a certain fungus that Alexander Fleming found tucked inside some molding plates in his lab in 1928. Antibiotics work because they attack something that distinguishes a microbial cell from the host (human) cell. Penicillin, for example, kills bacterial enzymes that synthesize the cell wall, testing in bacteria with “holes” in their walls. Human cells don’t possess these particular kinds of cellular walls. More on antibiotics in The Body by Bill Bryson.
- Quote (P. 67): “Every potent antibiotic — doxycycline, rifampin, levofloxacin —recognizes some molecular component of human cells that is different from a bacteria cell. In this sense, every antibiotic is a ‘cellular medicine’ — a drug that relies on the distinctions between a microbial cell and a human cell. The more we learn about cell biology, the subtler distinctions we uncover, and the more potent antimicrobials we can learn to create.”
- Three Classifications of Cells — Every cell on Earth, which is to say every living being, belongs to one of three branches of living organisms. In other words, all cells, which make up every living organism on the planet, fall into one of three buckets. These buckets are:
- Bacteria — This chapter has been all about bacteria/microbes. Bacteria are living organisms that have one cell and possess unique characteristics. These unique characteristics distinguish bacterial cells from human cells and are what antibiotics target when trying to kill bad bacteria that is causing disease. We have “several billion” bacteria/bacteria cells on us at all times, mostly good/non-violent bacteria. These good bacteria aid in digestion, help our skin, and do a lot of other great things for us. Only some kinds of bacteria are considered bad and can cause disease, especially when they start to reproduce. It’s amazing how many bacteria cells are on us.
- Quote (P. 68): “An infectious disease specialist once told me that humans were just ‘nice-looking luggage to carry bacteria around the world.’ He might have been right.”
- Quote (P. 68): “The abundance and resilience of bacteria stagger the mind. Some live in oceanic thermal vents where the water reaches near boiling temperature; they could easily thrive inside a steaming kettle. Some prosper within stomach acid. Yet others live, with seemingly equal ease, in the coldest places on earth, where the land freezes into packed, impenetrable tundra for ten months of the year. They are autonomous, mobile, communicative, and reproductive.”
- Eukaryotes — Eukaryotes represent the second bucket that all cells (living organisms) fall into. Humans fall into this category. Cells that fall in this category have a nucleus. Bacteria don’t have a nucleus.
- Archaea — Archaea represent the third bucket that all cells (living organisms) fall into. Remarkably, archaea weren’t discovered and taken seriously until the 1970s. We still don’t know a whole lot about this category.
- Bacteria — This chapter has been all about bacteria/microbes. Bacteria are living organisms that have one cell and possess unique characteristics. These unique characteristics distinguish bacterial cells from human cells and are what antibiotics target when trying to kill bad bacteria that is causing disease. We have “several billion” bacteria/bacteria cells on us at all times, mostly good/non-violent bacteria. These good bacteria aid in digestion, help our skin, and do a lot of other great things for us. Only some kinds of bacteria are considered bad and can cause disease, especially when they start to reproduce. It’s amazing how many bacteria cells are on us.
- Chapter Takeaway — Microbes/bacteria are single-celled living organisms that live on our body. We have billions of them on us at all times, and only some of them are harmful. Bad microbes essentially cause disease by invading us and interrupting our human cells. This is different than a disease like cancer, which occurs when our human cells rebel against us. Antibiotics were discovered in the early 1900s and help kill bad bacteria when we’re experiencing an illness caused by bad microbes.
Ch. 5: The Organized Cell
- The Three Properties of Cells — The three properties that define a cell are autonomy, reproduction, and development. These properties allow cells to make up an organism’s body and function correctly. A look at these three:
- Autonomy — To survive as an independent living unit, cells have to be autonomous, meaning they can act independently. This autonomy depends on the cell’s interior anatomy. A cell is not a blob of chemicals; it has distinct subunits within it that allow it to function independently. These subunits within cells are designed to supply energy, discard waste, store nutrients, deny toxic products, and more.
- Reproduction — Second, a cell is designed to reproduce, so that one cell can produce all the other cells that make up the organism’s internal parts and overall body.
- Development — Lastly, a cell must be able to develop into other specialized cells (e.g. liver cell, white blood cell, muscle cell), so that various parts of the body — tissues, organs, organ systems — can be formed. If a cell can’t develop into these other “specialized cells,” the organism can’t be fully created.
- A Tour Through a Cell — This chapter is primarily about the anatomy of a cell. What is inside of a cell? What do certain parts of the cell do? How do the different parts work together? Use the analogy of a human body; our bodies have different parts that carry out certain functions. The same goes for the inside of a cell. Below is a brief look at what makes up a cell:
- Cell Membrane — The cell’s membrane is its outer shell. It’s the boundary. For a human body, the membrane is the skin. The cell membrane isn’t sealed shut; it has pores that allow certain key nutrients inside. These pores also allow the cell to get rid of waste and toxins. This is necessary for survival. Unfortunately, having openings also makes the cell vulnerable to allowing viruses and bad bacteria inside.
- Cell Membrane Surface — The cell membrane is made up of two layers of lipids, meaning it’s double sealed. Imagine a balloon wrapped in two sheets of paper. The paper is the cell membrane’s surface. Floating on the membrane’s surface are proteins that act as receptors, signals, and glue for attaching to other cells.
- Internal Fluid — A cell is filled with internal fluid. This internal fluid is called protoplasm. Protoplasm is thick in some areas; watery in others. It’s kind of like jelly.
- Skeleton — Just like a human has a skeleton that helps it maintain its form, cells have something called a cytoskeleton that forms its overall structure. The cytoskeleton is critical for cell movement; cells would not be able to move and swing around without the cytoskeleton.
- Nucleus — This is the most important part of the cell. Every human, animal, and plant cell has a nucleus. Bacteria don’t. The nucleus is where the bulk of the cell’s genetic code, the instruction manual for life, is stored. It is the storage bank for DNA. RNA (next), the code for building proteins, is created in the nucleus then exported out into the cell to help build proteins.
- RNA — RNA is a critical molecule found inside the cell. The molecule is long and looks like a strand. An RNA strand carries the message, or code, to build a protein. It’s a set of instructions for building proteins. One particular RNA, freshly made in the cell’s nucleus, may arrive carrying the instructions to build, for example, insulin.
- Ribosomes — Ribosomes capture RNAs and decode their instructions for making proteins. Ribosomes are everywhere inside a cell; liver cells contain several million of them.
- Proteins — There are tons of proteins floating in the protoplasm. Proteins are what RNA molecules make via their decoding mechanisms. These proteins make possible many living reactions, like respiration, metabolism, and disposal. Building proteins is one of the cells major tasks. Proteins form enzymes that control the chemical reactions of life. They create structural components of the cell. They are the receptors for signals from the outside. They form pores and channels across the membrane, and the regulators that switch genes on and off in response to stimuli. Proteins are the workhorses of the cell.
- Mitochondria — Mitochondria are kidney-shaped organelles that serve as the fuel generators of the cell. They glow and burn constantly to produce the energy needed for life. They are in all cells, but they are really packed in cells that need the most energy, such as muscle cells, fat cells, and certain brain cells. Again, the mitochondria are where most ATP (energy) is created in the cell. Mitochondria produce energy.
- Endoplasmic Reticulum (ER) — The ER is a winding stairway found in almost every cell. The ER is where proteins inside the cell are synthesized and exported. The ER’s role is crucial because proteins, in turn, carry out all work in the cell.
- Golgi — The Golgi is where most proteins in the cell go when they make it through the ER. It’s a specialized compartment where proteins are stored. From the Golgi, proteins are transported out of the cell.
- Secretory Granules — These are the transportation vehicles within a cell that take proteins from the Golgi and export them out of the cell.
- More on the Nucleus & DNA — Back to the nucleus, which is the most important part of the cell and where all of our DNA is stored. Every cell contains about six feet of DNA, if the DNA was to be stretched out from its tight packaging. Collectively, the DNA (genes) from our 36 trillion cells would stretch to the sun and back 60 times. Within a cell, proteins, as well as hormones attached to proteins, can travel in and out of the nucleus, turning genes within our DNA on and off. This is crucial because our DNA/genes are what give cells their identity (e.g. “be a white blood cell”; “be muscle cell”).
- How Cells Create Proteins — Building on the definitions of cell parts outlined above, the whole process of creating proteins inside a cell can be imagined as a postal system. It begins with the code of genes (RNA) that is translated to write the letter (the protein). The protein is written, or synthesized, by the cell’s letter writer (the ribosome), which then posts it to the mailbox (the pore by which the protein enters the ER). The pore routes it to the central posting station (the ER), which then sends the letter to the sorting system (the Golgi), and finally brings it to the delivery vehicle (the secretory granule). This “postal system” is how most proteins get to their correct locations within the cell.
- How Cells Generate Energy (ATP) — Our cells create energy for us. There are two main ways a cell generates energy: a fast method and a slow method. Both processes result in the creation of adenosine triphosphate, or ATP. ATP is energy. It is the central currency of energy in all living cells. Any chemical or physical activity that requires energy — for instance, the contraction of a muscle or the synthesis of a protein — utilizes, or “burns,” ATP. Over the course of a day, we generate billions of little canisters of fuel (ATP) that fire a billion little engines inside the billions of cells in our body. Without energy, we are dead. Creating ATP is one of the ways our cells keep us alive.
- Fast Way — The fast way occurs in the cell’s protoplasm via enzymes breaking glucose into smaller and smaller molecules. This reaction produces energy (ATP).
- Slow Way — The slow way takes place in the cell’s mitochondria and involves a slow burn of sugars. It generates a much larger amount of energy (again, in the form of ATP).
- Chapter Takeaway — Just like the human body has internal parts that perform certain functions, every one of our 37 trillion cells has a whole world of activities going on inside. It’s like a body inside of a body. Two important things every cell is trying to accomplish include creating energy (in the form of ATP) and building proteins, which then carry out most of the work in the cell. Every human, animal, and plant cell has a nucleus, which is where our DNA is stored.
Ch. 6: The Dividing Cell
- Cell Division: Two Kinds — Other than some cells, like certain neurons, all cells divide. And there are two reasons for why they divide: production (mitosis) and reproduction (meiosis). A brief look at both of these.
- Production (Mitosis) — Production refers to the creation of new cells to build, grow, or repair an organism. When skin cells divide to heal a wound, when T cells divide to produce an immune response, the cells are giving birth to new cells either to produce a tissue or an organ, or to fulfill a function.
- Reproduction (Meiosis) — The other reason why cells divide is for reproductive purposes. This process describes how sperm or eggs are generated in the human body. Here they are being generated to undergo reproduction — dividing to produce a new organism rather than a new function or an organ.
- Chromosomes & DNA — To understand how and why a cell divides, it’s important to first know that each cell has chromosomes, and these chromosomes are where DNA molecules are located. DNA carries all genetic information, therefore genetic information is stored in a cell’s chromosomes. This is important because one of the big events that occurs during cell division is the splitting of chromosomes into a new cell.
- How a Cell Divides: Production (Mitosis) — When a cell divides for production (mitosis) purposes, the cell’s chromosomes (located in the nucleus) change physiology, going from being loose threads to tightening into a bundle. The nuclear membrane then dissolves, and the chromosomes separate into two sides of the cell. When they’re fully separated, they split, producing two cells. See the image below for a look at this process. It’s important to note that human cells always have 46 chromosomes during mitosis — they start with 46, then double to 92. As they double, the two bundles of chromosomes move away from each other within the cell. When they’ve stretched far enough away from each other, they divide, with each splitting cell ending up with 46 chromosomes.
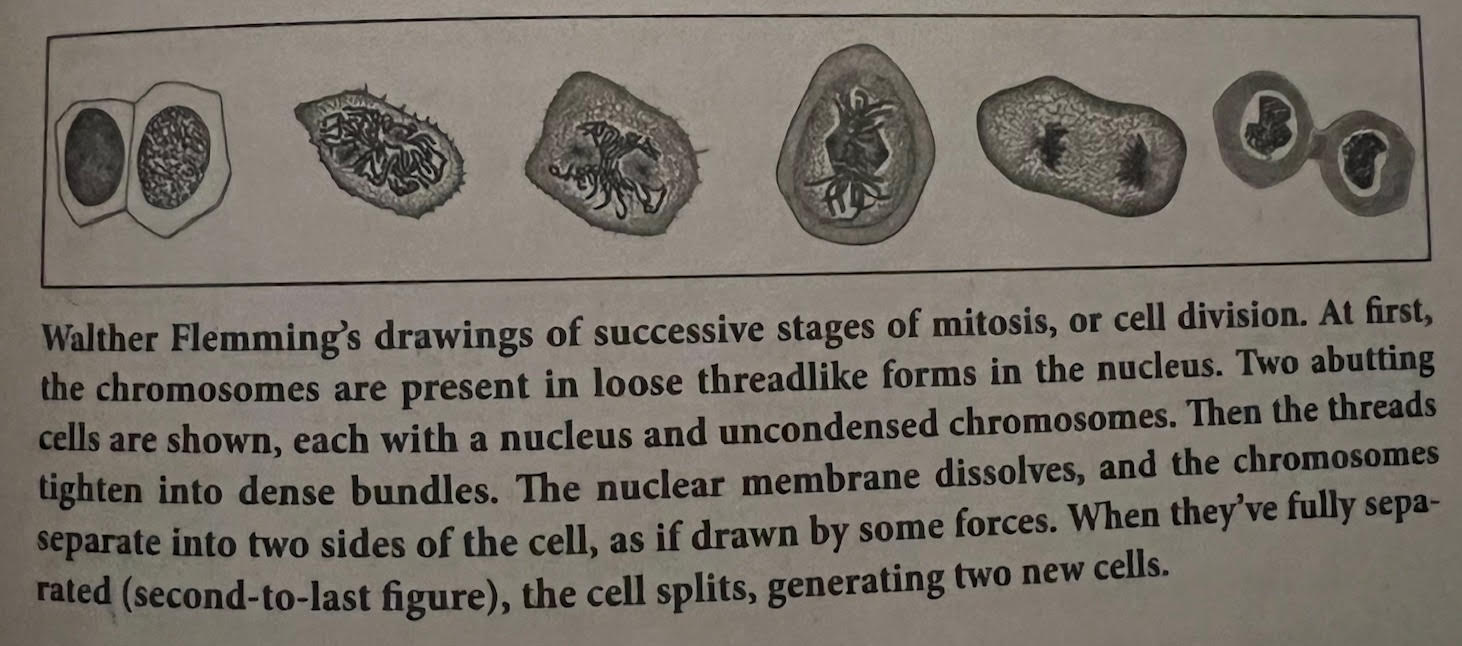
- How a Cell Divides: Reproduction (Meiosis) — The process is a little different when a cell is undergoing the reproductive process (meiosis). Rather than 46 chromosomes, sperm cells in the testes (men) and egg cells in the ovaries (women) have 23 chromosomes each, which turn into 46 when the sperm cell meets the egg cell and fertilizes to create a zygote.
- The Cell Division Cycle: Mitosis and Meiosis — In short, the life cycle for a multicellular organism is essentially a back-and-forth game between meiosis and mitosis. Humans, starting with 46 chromosomes in every bodily cell, produce sperm cells in the testes and egg cells in the ovaries via meiosis, each ending up with 23 chromosomes. When sperm and egg meet to form a zygote, the number of chromosomes is restored to 46. The zygote grows through cell division, mitosis, to produce the embryo, and then develops progressively mature tissues and organs — heart, lungs, blood, kidneys, brain — with cells that have 46 chromosomes each.
- In Vitro Fertilization (IVF) — IVF is the joining of a woman’s egg and a man’s sperm in a laboratory dish. “In vitro” means outside the body. IVF is a form of cell therapy; one of the most common cell therapies in human use. It’s been a reproductive option for more than 40 years and has produced 8-10 million children. IVF was invented to help solve infertility. The techniques of IVF are pretty wide-ranging, but the practice usually involves fertilizing sperm and eggs outside the body (in a lab) to create a living human embryo, then implanting it into a female womb to produce a baby. These are often called “test tube babies” and help couples who are struggling with infertility. The first IVF baby was Louise Brown, born in 1978.
- Chapter Takeaway — Cells divide for two reasons: production (mitosis) and reproduction (meiosis). When cells divide for production purposes, they are creating new tissues and organs (e.g. creating a human), or fulfilling some kind of function (e.g. skin cells dividing to heal a wound). When cells are dividing for reproductive purposes, they are doing so to create a new organism (e.g. baby).
Ch. 7: The Tampered Cell
- Gene Editing — Building on IVF, scientists have found a way to edit genes in the embryo phase (after the eggs and sperm have been fertilized in a dish). The idea behind this is to detect abnormalities in an embryo’s chromosomes (again, where DNA is stored in a cell) and selectively remove them before implanting the embryo in the mother’s womb. As a result, a couple would be able to avoid implanting an embryo that has genetic problems in its chromosomes like Down Syndrome or cystic fibrosis, for example.
- Gene Altering: Early Success — Altering genes first experienced success in the 1960s, when Robert Edwards was able to snip certain cells and chromosomes from rabbit embryos to guarantee the sex (male or female) of a rabbit’s offspring. Edwards’s success opened the door to new possibilities. If we can control the sex of an animal’s offspring, what other genes can we alter? Scientists have been experimenting for decades, trying to figure out ways to cut certain genetic problems out of DNA in the embryo phase in order to prevent offspring from inheriting lethal diseases and syndromes. Once the genetic edits have been made, then the embryo is implanted into the mother’s womb.
- Gene Editing: Cas9 Protein — Although scientists like Edwards had success altering genes in certain animals, the process is far more difficult in humans. As a result, human gene editing has not happened yet. But in 2011, a new gene editing method was introduced that is changing the game. It involves a bacterial protein called Cas9. Basically, Cas9 is introduced into human cells and then “guided” to a specific part of a cell’s genome (via a strand of RNA in the cell) to make deliberate edits. These edits are made by making a “cut” in the genome that disables the targeted gene. Bacteria use this system to chop up the genes of invading viruses in the body, thereby disabling the invader. Scientists are trying to use the system via Cas9 to make purposeful cuts in the genome.
- Cas9: How It Works — To further understand how Cas9 works, it’s good to use an analogy. Imagine the human genome as a huge library. The books are written in an alphabet with four letters: A, C, G, and T — the four building-block chemicals of DNA. Reframed as a library of books, with about 250 words per page and 300 pages per book, we might think of ourselves — or rather the instructions to build, maintain, and repair ourselves — as written in about 80,000 books. Cas9, when combined with a piece of RNA to guide it, can be directed to make a deliberate change in the human genome. Sticking with the analogy, it can be used to find and erase one word in one sentence on one page in one volume of that 80,000-book library. It’s a search and destroy eraser.
- Future of Gene Editing — Whether by sex selection or by gene editing, the genetic manipulation of the human embryo to remove diseases (or, perhaps, to enhance human abilities) seems to be something that will become commonplace in medicine very soon. There are a lot of ethical concerns with gene editing. Permanently altered genes in one child will later be passed down to the child’s child. It’s critical to get this right. By editing genes in the embryo phase, you’re messing with somebody’s life before it even gets started.
- Quote (P. 130): “But whether by embryo selection or by gene editing, the genetic manipulation of the human embryo to arrest diseases (or, perhaps, to enhance human abilities) seems, every day, to become an inevitable destination for medicine.”
- Chapter Takeaway — Gene editing in the embryo phase, either to remove the possibility of lethal diseases or enhance humans, is coming very soon. Scientists have made huge advances in this area, and the use of the Cas9 bacterial protein is only speeding things up. By introducing Cas9 into our cells and guiding it to the correct locations, scientists can make deliberate edits (or “cuts”) to the human genome.
Ch. 8: The Developing Cell
- Making a Baby — The process by which a baby is born is very complex and interesting. Everything starts with one cell (when one sperm cell meets one egg cell, forming a human zygote). From there, the cell divides rapidly to form all of the various organs and systems inside the baby. The process can be described in the following phases:
- Zygote Is Formed — After mom and dad do their thing, sperm swim through the woman’s body until one sperm cell penetrates an egg. A special protein on the surface of the egg and the receptor on the sperm clicks the two cells together. Once attached, a wave of ions diffuses in the egg, which prevents other sperm from entering. The human zygote (a one-cell organism) is formed.
- Blastocyst, Embryo, and Fetus — From here, the fertilized cell divides into two, then two into four, and so forth, until a small ball of cells is formed. This ball of cells is called a blastocyst and resembles a water balloon with a fluid center. As cells keep dividing and developing, the unit becomes an embryo. Later, it becomes a bat-like lump of cells hanging inside the ball/cellular balloon, called a fetus.
- Ectoderm, Endoderm, and Mesoderm — Next, the tiny cluster of cells hanging from the walls of the cellular balloon divides furiously and begins to form two layers of cells — the outer one called the ectoderm, and the inner called the endoderm. And about three weeks after conception, a third layer of cells invades the two layers and lodges itself between them, like a child squeezing into bed between her parents. It’s now the middle layer, called the mesoderm. These three layers help create every organ in the body.
- Quote (P. 139): “This three-layered embryo — ectoderm, mesoderm, endoderm — is the basis of every organ in the human body. The ectoderm will give rise to everything that faces the outer surface of the body: skin, hair, nails, teeth, even the lens of the eye. The endoderm produces everything that faces the inner surface of the body, such as the intestines and the lungs. The mesoderm handles everything in the middle: muscle, bone, blood, heart.”
- The Notochord — The embryo is now ready for the final sequence of activities. Within the mesoderm, a series of cells assemble to form a rodlike structure called the notochord, which spans from the front of the embryo to its back. The notochord will become the GPS of the developing embryo, determining the position of the internal organs. In response, just above the notochord, a section of the ectoderm — the outer layer — invaginates, folding inward and forming a tube. This tube will become the precursor of the nervous system, made up of the brain, spinal cord, and nerves.
- Organs Begin to Form — Once the notochord and the neural tube have been generated, individual organs begin to the form out of the three layers (four, if you count the neural tube): the heart, the liver, the intestines, the kidneys. About three weeks later, the heart will generate its first beat. A week after that, one part of the neural tube will begin to form the brain. All of this, remember, emerged from a single cell: the fertilized egg. Below is a look at parts of the process.
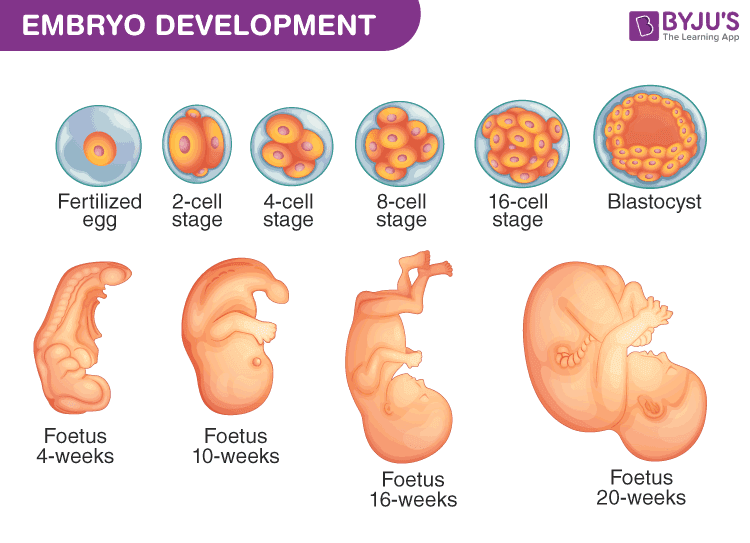
- How an Embryo Grows — The steps outlined above are a description of the baby making process, but they don’t touch on how cells know what to do and when to do it. It’s an extremely complicated process, but at a basic level it can be described as such: At each stage, cells that have already been created release proteins and chemicals that serve as signals, telling newly-created cells where to go and what to do. These cells lead the formation of layers, as well as tissues and organs. The cells within these layers turn genes on and off depending on the type of cell it is (e.g. red blood cell, muscle cell, heart cell, etc.). For example, a heart cell won’t need to do everything a skin cell needs to do, so certain genes are turned on and off based on the cell’s identity. In this way, with preexisting cells directing new cells, each stage of the embryonic process builds on the prior one.
- Quote (P. 142): “The growth of an embryo is a process, a cascade. At each stage, preexisting cells release proteins and chemicals that tell the newly emerging and newly migrating cells where to go and what to become. They command the formation of other layers and, later, the formation of tissues and organs. And the cells within these layers themselves turn genes on and off, in response to location and their intrinsic properties, to obtain their self-identities. One stage builds upon signals emerging from a prior stage. . . This is how we build our cellular house.”
- Quote (P. 145): “As the zygote develops, its cells need to determine their identities and positions by integrating extrinsic factors (proteins and chemicals coming from neighboring cells that signal a cell telling it where to go and what to become) and intrinsic factors (proteins in the cell, encoded by genes, that are turned on and off in response to these signals).”
- The Saga of Thalidomide — In the 1960s, a German company developed an anxiety drug called Thalidomide that was prescribed to tens of thousands of women going through pregnancy. Frances Kelsey of the FDA later won a Presidential Medal of Honor for her role in preventing the drug from coming into the U.S. Why? By degrading proteins inside of the cells of an embryo, Thalidomide messed up the signals between cells as the embryo was growing, leading to miscarriages and deformed babies. As the previous bullet touched on, these signals are critical because they direct new cells and tell them where to go and what to do. By interfering with these signals, Thalidomide caused serious harm; many babies were born with short limbs or died in the womb.
- Quote (P. 145): “Thalidomide, we now know, binds to one (or several) of the proteins in the cell that break down other specific proteins; it acts as a protein-specific degrader: An intracellular protein eraser. . . Unable to integrate the signals that they receive, the cells likely die or become dysfunctional.”
- Chapter Takeaway — The embryo development process starts when a sperm cell penetrates an egg cell and becomes a human zygote (single-celled organism). From there, the cell divides once, then twice, then many more times, eventually building into a multi-cellular fetus. How cells know where to go and what function to carry out during the process is complex. At a basic level, cells that come earlier in the process lead and direct new cells by releasing signals via proteins and chemicals. These signals tell new cells where to go and what to do. That’s why the drug Thalidomide was so bad for women who were in pregnancy — it degraded a cell’s proteins, which, in turn, messed up the communication and signaling between cells, leading to cellular death or malfunction.
Ch. 9: The Restless Cell
- Organs — Every organ in the body is made up of cells and has the features such as self-defense, self-recognition, digestion, metabolism, storage, and waste disposal. The way organs are able to provide these functions is by working cooperatively between cells and through cellular specialization. In other words, the cells that make up all of our organs specialize in what they do, but they also work together with each other and other cells in the body to execute all of the different things an organ needs to do to function correctly.
- Blood — Blood is a great example of how an entire system of cells in the body works together to achieve its functions. The constant circulation of blood works as the body’s central highway to deliver oxygen and nutrients to all tissues. It ensures a coordinated response to injury: the process of blood clotting uses the circulatory system to slow or stop bleeding and to help wounds heal. And it also enables a response to infection: white blood cells use the same central highway to look for and fight pathogens (e.g. diseases, influenza, viruses, etc.).
- Blood Is an Organ — It’s not often considered as such because it moves freely around the body, but blood is an organ. It’s a living, breathing thing. When blood leaves the body, it degrades quickly, just like other organs. That’s why it has to be treated so carefully when drawn from somebody; if it spends much time in free air outside the body, it becomes an unusable clog of gel.
- Blood: A Communication System — Blood is a network. Whether it’s hormones, nutrients, oxygen, or waste products, blood delivers resources to every organ in the body. Blood also acts as a communication system in the body; it talks to every organ and tissue. There are four main components of blood:
- Platelets — Platelets band together to form a clot. Clotting is designed to stop bleeding. As soon as a bleed starts, millions of platelets begin to cluster around the wound and deposit material called fibrin. Together, these form a plug to stop the bleeding.
- Red Blood Cells — Red blood cells are designed to do one job: deliver oxygen to our other cells throughout the body. A teaspoon of human blood contains about 25 billion red blood cells. Red blood cells are almost entirely hemoglobin, which is the most important part of a red blood cell. Hemoglobin is a protein in red blood cells that carries oxygen; it’s basically a shipping container for the oxygen.
- White Blood Cells — White blood cells have the most complex job of all: they signal one another to coordinate an immune response; they facilitate the healing of wounds; and they fight microbes and survey the body for invaders.
- Plasma — Plasma is the fluid component of blood. Although 90% of plasma is water, it carries other important materials like carbon dioxide, hormones, waste products, nutrients, clotting factors, and chemical signals.
- Blood: Running Through the Body — A typical red blood cell will be shot around the body about 150,000 times before it is too battered to go on. At that point, it is sent to the spleen to be discarded. Discarded red blood cells are part of the reason why poop is brown and pee is yellow. The heart pumps blood around the body in a continuous circle. Blood enters the heart, leaves it, then returns to the heart after it has completed its circle around the body.
- Red Blood Cells: Oxygen Vehicles — Red blood cells are the most plentiful cells in the blood and are almost entirely made of hemoglobin, a protein that carries iron. Attached to iron is oxygen. All of this means that the primary function of red blood cells is to carry oxygen, which comes attached to hemoglobin via iron, to all tissues and organs in the body. This is what red blood cells are doing when they circulate the body constantly; they pick up oxygen in the lungs and carry it to the heart, which then shoots the cells out into the rest of the body so they can deliver oxygen.
- Quote (P. 156): “By the late 1880s, however, physiologists had begun to understand the importance of the ‘glob.’ They noted that hemoglobin carried iron, and the iron, in turn, bound oxygen, the molecule responsible for cellular respiration. . . The principal purpose of the red blood cell was to ferry oxygen, bound to hemoglobin, to tissues in all the body’s organs. Red cells pick up oxygen in the lungs, then are routed to the heart, which propels them on their voyage through the arteries to the rest of the body.”
- Quote (P. 375): “Hemoglobin is blood’s hidden secret — a complex of proteins so vital to our existence as organisms that we have evolved a cell [red blood cell] whose principal job is to act as a suitcase to carry it around.”
- Blood Transfusions — Blood transfusion, the transfer of blood from one person to another, was the first modern form of cell therapy. The procedure’s rapid development in the early 1900s changed medicine significantly and has been a major factor in surgeries, anemia treatment, cancer chemotherapy, trauma medicine, bone marrow transplantation, safety in childbirth, and much more. It is involved in so many different medical procedures.
- Quote (P. 162): “It is virtually impossible to envision the development of modern surgery, safe childbirth, or cancer chemotherapy without the invention of blood transfusion.”
- Weird History of Bleeding — What’s interesting is that people used to think bleeding somebody was the best way to cure them of a disease. George Washington, for example, died after being bled in response to a sore throat. He lost 40% of his blood before passing away.
- Four Blood Types — In 1900, Karl Landsteiner led the charge in making blood transfusions safe and usable. By 1907, doctors used his methods to perform the first safe blood transfusions between humans. His findings changed medicine forever, and he later won a Nobel Prize for his work in 1930. Landsteiner essentially mixed and matched blood from different donors in his lab and discovered that there are four main blood types. These groups indicate transfusion capability, meaning blood types between a donor and recipient should be a match before attempting to transfuse blood. The four blood types are:
- Type A
- Type B
- Type AB
- Type 0
- Quote (P. 159): “In a single table, Landsteiner delineated the four basic blood groups and laid the basis for blood transfusion. It was an advance of such medical and biological significance that that one table alone would be sufficient to award Landsteiner the 1930 Nobel Prize in Physiology or Medicine.”
- How Blood Types Work — The way blood typing works is this: All blood cells are the same inside, but the outsides are covered with different kinds of antigens — proteins that project outward from the cell surface — and that is what accounts for the four blood types above. People with blood type A can donate to those with A or AB but not B; people with B can donate to B or AB but not A; people with AB can donate only to other people with AB blood. People with type O blood can donate to all others, and are known as universal donors. Type A cells have A antigen on their surface, type B have B, and type AB have both A and B. Put A type blood in a B type person and the recipient body sees it as an invasion and attacks the new blood.
- Blood Transfusion in War — The development of blood transfusion came at the perfect time; not long after the procedure became commonplace, World War I and World War II broke out. Soldiers were getting torn apart and needed blood on the battlefield. War doctors knew how to match and transfuse blood, which saved a lot of lives.
- Chapter Takeaway — Blood is a living, breathing thing. It is an organ. About 90% of a red blood cell’s weight consists of hemoglobin, a protein that carries iron, which comes with oxygen attached. As a result, the primary job red blood cells have involves ferrying oxygen to all of our organs and tissues. A typical red blood cell will be shot around the body about 150,000 times before it is too battered to go on. White blood cells help fight infections, and platelets band together to form a clot and stop bleeding.
Ch. 10: The Healing Cell
- Platelets — Platelets are fragments of cells that float in the blood and are responsible for clotting in response to a cut or wound. Platelets are one of the four main components of blood discussed in the previous chapter. In appearance, they look like flat, round, plate-like shards. The primary role that platelets serve is to control bleeding when there’s an injury. When you have a cut, swarms of platelets rush to the injury site and clot in order to stop the bleeding. Skin cells then divide until they touch each other in order to stitch the wound up.
- Quote (P. 165): “Rather than possessing a single nucleus, it [platelet] was a cell with more than a dozen nuclear lobes. It had been born, presumably, from a mother cell that had replicated its nuclear contents but halted the division or birth of daughter cells — preferring, instead, to mature and then splinter into a thousand fragments. Indeed, as Wright followed the fate of these megakaryocytes (massive, multi-nuclear-lobe-carrying cells), he found that they broke up, like fireworks, into thousands of little shards — platelets.”
- Quote (P. 165): “As Bizzozero had observed, platelets were found to be the central component of a clot. Activated by signals from an injury — a wound, say, or a broken blood vessel — they swarmed to the wound site and began a self-perpetuating loop to plug the bleeding. It was a healing cell (or, more accurately, cell fragment).”
- Platelets: Clotting Gone Wrong — Although platelets serve a tremendous role for us by plugging up cuts at the source and stopping the flow of bleeding, they have become dangerous because of our modern lifestyle. Because we sit around more, live longer lives, don’t exercise enough, smoke, and have a lot of fat in our diet and around our waist, plaque builds up in our arteries. When a plaque ruptures or breaks, it is sensed as an injury, prompting platelets to rush to the injury site to plug it up. The problem is that when the platelets come over to clot the wound, they plug up our coronary arteries, causing a blockage and preventing blood from reaching the heart. The result is a heart attack.
- Quote (P. 167): “And so, as much as ancient humans may have desired a drug to activate platelets to heal their wounds, modern humans are in search of drugs that dampen platelet activity. Our lifestyles, lifespans, habits, and environments — fat-rich diets, lack of exercise, diabetes, obesity, hypertension, and smoking, in particular — have led, in turn, to the accumulation of plaques: inflamed, calcified, cholesterol-rich blobs that hang on the walls of the arteries, like precarious mounds of debris alongside highways, accidents waiting to happen. When a plaque ruptures or breaks, it is sensed as a wound. And the ancient cascade to heal wounds is activated and released. Platelets rush in to plug that ‘wound’ — except the plug, rather than sealing an injury, blocks the vital flow of blood into the heart muscle. The healing platelet now turns into a deadly platelet.”
- Interesting Fact — Until the early 1900s, the condition we now recognize as a heart attack wasn’t really well known in the medical world. When somebody dropped over dead one day, nobody really knew exactly why. In 1912, a cardiologist in Chicago described the mysterious case of a 55-year-old banker who “fell like a dud.” As doctors investigated the case, they found that the artery that brought blood to the patient’s heart had become blocked by a clot. The condition became commonly known as a “heart attack” from that point forward.
- How Plaques Form — It generally takes decades for plaque to build in the arteries. Peter Attia’s book Outlive discusses this. But at a basic level, plaque forms when levels of a cholesterol-rich particle called low-density lipoprotein (LDL) are high in the blood. Normal cells in the body carry receptors designed to collect and metabolize LDL, allowing the cells to remove these particles from the blood stream, keep LDL levels low, and therefore prevent plaque buildup. But when LDL levels are high for whatever reason (genetic problems preventing cells from metabolizing LDL, an older body, poor lifestyle habits, etc.), plaque builds and heart attacks can happen due to platelets clogging key arteries. These findings in the 1960s led to the development of cholesterol-lowering drugs like Lipitor that have reduced plaque buildup and had a huge impact on cardiac disease. The development of these drugs has saved millions of lives.
- Aspirin: A Heart Attack Preventer — As the death toll from heart attacks mounted in the 1950s and 1960s, pharmacologists shifted their attention to finding drugs that prevented the problem of clotting. The most prominent among these was aspirin. Its active ingredient, salicylic acid, originally found in willow extract, had been used by the ancient Greeks, Sumerians, Indians, and Egyptians to control inflammation, pain, and fever.
- Aspirin: How it Works — How does aspirin work to prevent heart attacks? Researchers in the 1960s found that platelets, in concert with some other cells, produce chemicals to signal injury and get activated. Aspirin blocks the key enzyme that produces these injury-sensing chemicals, thereby decreasing platelet activation and subsequent clots. In short, aspirin prevents clotting by blocking platelets from giving off signals that there’s an injury in the body. This, in turn, prevents clotting from taking place in the arteries.
- Quote (P. 169): “As a prevention mechanism for heart attacks, aspirin may well rank among the most important medicines of the past century.”
- Origins of Aspirin — Aspirin’s development dates back to the late 1800s. In 1897, a chemist named Felix Hoffman, working for the German pharmaceutical company Bayer, found a way to synthesize a chemical variant of salicylic acid. The medicine was later called aspirin, or ASA, short for acetyl salicylic acid. In the 1940s and 1950s, Lawrence Craven, a practitioner in California, began giving patients aspirin to prevent heart attacks. His study was dismissed for decades until, in the 70s and 80s, massive randomized trials “proved that aspirin was indeed among the most effective therapies to prevent and treat a heart attack in progress.”
- Heart Attacks: How They Happen — The chapter has already touched on this, but heart attacks occur when a plaque in one of the coronary arteries ruptures and causes platelets to rush to the scene to clog the wound. Blood, which carries vital oxygen, is then unable to reach the heart because the artery is blocked. When this happens, it is critical to get medical attention as fast as possible; every minute wasted is a minute’s worth of heart muscle dying. At the hospital, the patient is usually given a drug known as a thrombolytic, which can dissolve a blood clot rapidly. Or a doctor can try to open the clotted artery using an inflatable, balloon-shaped device. The key is to somehow get the artery unlocked so blood flow can return to the heart.
- Arteries: “The Rivers of Life” — A good way to understand how heart attacks work involves picturing the coronary arteries as rivers. Like a growing collection of trash on the edge of a river, coronary plaque usually builds up over decades, inching out toward the center of the artery and slowing down blood flow, although never obstructing it entirely. The artery’s opening is narrowed by the plaque (trash), resulting in chest pains, which are a sign that blood is having a hard time keeping getting to the heart. One day, the trash (plaque) spills into the river. Platelets, the body’s injury detectives, rush into the injury site to plug it up. The slowed-down traffic in the river now turns to a standstill jam — a heart attack.
- Drugs to Address Heart Health — Over the years, pharmacologists have discovered many drugs and procedures to prevent or treat heart attacks. There’s aspirin, which prevents platelets from forming clots. There are clot-dissolving drugs that break up an active clot, and platelet-inhibiting drugs that ensure that platelets don’t get activated. And, when it comes to prevention, there’s Lipitor, one of the many drugs that reduce the level of LDL cholesterol in the blood. Drugs like Lipitor decrease the blood levels of LDL, and that, in turn, prevents the buildup of cholesterol-rich plaque to clog our arteries.
- Heart Attacks & Gene Editing — There are doctors currently working on a way to edit genes inside of liver cells that regulate the production of LDL cholesterol. Some people have liver cells that produce too much LDL cholesterol, which leads to a buildup of plaque in the arteries. This gene-editing enzyme would be delivered via a catheter into the liver, where it would “turn off” certain genes in liver cells that produce bad cholesterol, leading to permanently lower LDL levels in the blood.
- Chapter Takeaway — Heart attacks happen when a piece of plaque inside our arteries ruptures or breaks, causing platelets in the blood to race over to clog the wound. When the platelets clog the wound, they also clog the artery, which means blood cannot reach the heart. This results in a heart attack. Aspirin is a great heart attack aid because it prevents platelets from releasing signals that an injury has occurred. With the signal down, fewer platelets come to the injury site, which results in less clogging.
Ch. 11: The Guardian Cell
- White Blood Cells & The Immune System — White blood cells make up the cells in our immune system. These cells are responsible for detecting and fighting bacteria and viruses causing infection and inflammation in the body. As we will learn in this chapter, the immune system has several different “wards,” including an “innate” ward and an “adaptive” ward. There are different kinds of white blood cells in both of these wards that carry out different roles when fighting infections.
- Injury Signals: Chemokines and Cytokines — When there is infection or inflammation present in the body, injured cells call for help by releasing proteins called chemokines and cytokines. These proteins summon immune cells from the “innate immune system” (neutrophils and macrophages) to the scene, where they literally begin fighting the invader causing the infection or inflammation. The goal is to kill and eat the invader.
- Quote (P. 175): “In a series of papers published in the mid-1880s that would eventually win him the Nobel Prize, Metchnikoff used the German word ‘Kampf’, meaning ‘fight,’ ‘combat’ or ‘tussle’, to encapsulate the relationship between an organism and its invaders.”
- Phagocytosis: Eating Bad Microbes — When immune cells get to the scene of infection or inflammation in the body, they begin fighting the invader, usually by trying to kill and literally eat the infectious agent or irritant. This process of eating and digesting an invader is called phagocytosis. When immune cells kill and eat the invader, it leads to immunity from that infection for the whole organism.
- Quote (P. 175): “The immune cells, he found, did not merely travel to the sites of inflammation. They tried to ingest — eat — the infectious agent or irritant that had accumulated at the site. He called the phenomenon phagocytosis: the engulfment and consumption of an infectious agent by an immune cell.”
- Macrophages, Monocytes, & Neutrophils — The human versions of phagocytic cells are called macrophages, monocytes, and neutrophils. These white blood cells are among the very first to respond to injuries and infections in the human body. Neutrophils are produced in the bone marrow. When an infection arises, neutrophils come out of the bone marrow and into our blood circulation. Summoned by the release of chemokines and cytokines, they then fly to the scene of the infection and begin beating the crap out of the invaders. They are the first responders on the scene and later summon T cells and B cells. Macrophages and monocytes are the eaters; their job is to eat invaders.
- Quote (P. 175): “Neutrophils live for just a few days after entering the circulation. But what dramatic days! Incited by an infection, the cells mature from the bone marrow and flood into blood vessels, hot for combat, their faces granulated, their nuclei dilated a fleet of teenage soldiers deployed to battle. They have evolved special mechanisms to move quickly through tissues, squirming their way through blood vessels like contortionists. It is as if they are maniacally driven to reach sites of infection and inflammation — in part, because they so keenly perceive the gradient of cytokines and chemokines released by injury. They are lean, energetic, mobile machines built for immune attack. Professional killers — guardian cells — on a mission.”
- The “Innate” Immune System: Our First Responders — There are two wards that make up the immune system: the “innate immune system” and the “adaptive immune system.” The innate immune system is the one described in this chapter: it acts as a first responder when there’s an infection, sending neutrophils and macrophages to the scene. It is referred to as “innate” because it exists inherently in us, with no requirement to adapt to, or learn, any aspect of the microbe that caused the infection, unlike B cells and T cells in the “adaptive immune system.” Instead, neutrophils and macrophages have been trained to fight any bad bacteria (see next bullet). They are the very first immune cell responders on the scene of an infection. The “adaptive” ward of the immune system involves T cells and B cells; the innate ward doesn’t involve those. As neutrophils and macrophages are doing the early fighting, they summon T cells and B cells to the scene.
- Quote (P. 177): “The innate system is not just among the most ancient, but, being the first responder, the most crucial to our immunity. We associate immunity with B and T cells, or with antibodies, but without neutrophils and macrophages, we would meet the fate of the decomposing fly.”
- “We’ll Fight Anybody” — One of the astonishing things about “innate immune system” cells like macrophages and neutrophils is that they have receptors that recognize proteins and other chemicals found on the surface of some bacterial cells and viruses. What does this mean? It means that these immune cells are already prepared for combat before they even begin fighting a bacterial cell. After centuries of fighting various microbes, our immune cells have adapted to the point where they come pre-built with these receptors that help them fight viruses and bad bacteria. The key here is that the receptors have trained them to fight indiscriminately, meaning they’ll fight anyone — they don’t target a specific bad microbe. That’s what B cells and T cells in the “adaptive immune system” do. Those cells target specific bad microbes.
- Vaccination: Engaging the Innate Immune System — Vaccination is about helping the body achieve immunity from a virus. It works by introducing a virus at a low dose into the body to activate the immune system. Antibodies from B cells rush to the scene to fight and kill the virus, which allows those antibodies to gain a memory of how to beat the virus. This allows you to beat the real virus if you ever catch it. The process of vaccination involves all levels of the immune system, but it starts with the “innate immune system.” Before B cells and T cells are engaged, neutrophils and macrophages get to scene first to do the early fighting. Vaccination was another one of those discoveries that changed human health forever.
- Quote (P. 181): “But the under-appreciated fact of vaccination is that it is, at first, a manipulation of the innate immune system. Long before B and T cells appear on the scene, the first step in vaccination is the activation of the first-responder cells: macrophages, neutrophils, monocytes, and dendritic cells. It is these cells that pick up the inoculum. . . Then, through various signaling processes, including phagocytosis, they digest and process the inoculum to initiate the immune response.”
- Quote (P. 182): “Vaccination, more than any other form of medical intervention — more than antibiotics, or heart surgery, or any new drug — changed the face of human health. (A close contender might be safe childbirth.) Today there are vaccines against the deadliest of human pathogens: diphtheria, tetanus, mumps, measles, rubella. Vaccines have been devised to prevent infection by human papillomavirus (HPV), by far the major cause of cervical cancer.”
- Editing Immune Cells — Mukherjee and his team have been working on a form of cell therapy where they are attempting to create immune cells in the “innate immune system” that can target and eat certain bad cells, like cancer cells. These cells have been beefed up to be able to eat 10 times the normal amount, and 10 times faster. After being withdrawn from the body then altered in the lab, the cells are inserted back into the body to do their thing. So far, they have been very effective in rats, completely eating cancer inside the body. The therapy has been approved by the FDA, and in 2022 a cancer patient in Colorado was the first to try the experimental treatment. No results as of yet.
- Chapter Takeaway — There are two wards of the immune system: the “innate immune system” and the “adaptive immune system.” The innate system, and the neutrophils and macrophages inside this system, serve as our first responders when there is an infection or inflammation in the body. They get to the scene and begin the fighting. They then summon the B cells, T cells, and antibodies of the adaptive immune system.
Ch. 12: The Defending Cell
- Interesting Fact — More than 80,000 snake bites are reported every year in India, the most of any country in the world. Snake bites were extremely deadly in 17th century India because nobody knew how to treat them.
- The Adaptive Immune System — The adaptive immune system is the second ward of the immune system and involves key players such as B cells, T cells, and antibodies. These white blood cells are highly skilled and can target certain viruses and bacteria. Typically, these cells arrive on the scene of an infection or virus after the neutrophils and macrophages of the innate immune system get there.
- Antibodies & Antigens — Before getting into how B cells work, it’s important to know what antibodies and antigens are. Antibodies are actual bodies created by B cells in response to an antigen in the body. Once created by B cells and released into the blood, they then go fight the virus or microbe represented by the antigen. An antigen is some kind of disease, toxin, microbe, bacteria, or virus. An example would be the West Nile Virus. If you get bitten by a mosquito with this virus, the virus will show up in your body as an antigen, which will set off the immune system, including B cells.
- B Cells: How They Work — B cells are the white blood cells that make antibodies. We produce B cells in the bone marrow. Every B cell has one single receptor on its surface for a particular antigen. When an antigen (from the West Nile Virus, for example) enters the body, the B cell with the correct receptor for that antigen binds to it. After binding, the B cell becomes activated, meaning it grows big and begins to duplicate furiously to create more of these B cells with the same exact receptor. The army of B cells with the correct receptor now begin to release their receptors into the blood. These receptors ARE the antibodies. The antibodies, now released into the blood, then go out and hunt down the other antigens and kill them (more on that in the next bullet).
- Quote (P. 194): “When the connection is made, and a particular lymphocyte [B cell] with a particular receptor is brought into the presence of the particular antigen, one of the greatest small spectacles in nature occurs. The cell enlarges, begins making new DNA at a great rate and turns into what is termed, appropriately, a blast. It then begins dividing, replicating itself into a new colony of identical cells all labeled with the same receptor. In the end, the dominant B cell clones, displaying the ‘right’ receptor (the one that best binds the antigen) blast away, outgrowing all others. . . As Ehrlich had imagined back in 1891, these blasts now begin to secrete the receptor into the blood. Freed from the B cell’s membrane and now floating in the blood, the receptor ‘becomes’ the antibody. And when the antibody is bound to its target [antigen], it can summon a cascade of proteins to poison the microbe and can recruit macrophages to devour, or phagocytose, it.”
- Antibodies: How They Fight — After being created by B cells and released into the blood, antibodies — which have a ‘Y’ shaped head — go out and bind to all of the virus’s antigens. Once attached to an antigen, they work with other players in the immune system (T cells, macrophages, etc.) to kill the antigen, usually by poisoning it and then calling in macrophages to eat it.
- “Memory” B Cells — The reason we slowly build immunity to a certain virus, microbe, or toxin involves “memory” B cells. When B cells that have the correct receptor for a particular virus’s antigens become activated, they don’t simply go away after they’ve finished killing all of the virus’s antigens. Instead, they stick around in the body for a long time, kind of like an elite SWAT unit that hides out, waiting for the same antigens to enter the body again. Because these B cells have fought the virus before, they remember how to beat it. As soon as the virus enters, they recognize it, reactivate, multiply, and begin creating the antibodies needed to kill it. This is how memory B cells work. This is why you become more immune to a certain virus each time you get it.
- Quote (P. 194): “Decades later, researchers demonstrated that some of these activated B cells don’t simply peter out. They persist in the body in the form of memory cells. In Thomas’s words, ‘The new cluster [of cells stimulated by the antigen] is a memory, no less.’ Once the fulminant infection has ceased and the microbe cleared, some of these B cells become more quiescent, but they persist — finches huddled in the cave. When the body encounters the antigen again, the memory B cell is reactivated. It arises out of dormancy into active division to mature into an antibody-making plasma cell, thereby encoding an immunological memory.”
- Memory B Cells & Vaccination — Memory B cells are a big reason why vaccination is so effective. Vaccines are designed to introduce a virus or microbe and its antigens into the body at a low, safe dose in order to activate the immune system’s B cells. By activating B cells, which then create the needed antibodies to kill the virus, the vaccine is helping the body produce memory B cells that can spring into action immediately if you happen to get the real virus later on.
- Chapter Takeaway — B cells, by creating antibodies, are one of the important players in the “adaptive immune system.” When you have a virus or illness, antigens are released in the body. The B cell that has a receptor that matches the antigen binds to the antigen, which activates the B cell. It then gets big, multiplies, and releases its receptors (antibodies) into the blood. Antibodies then go out and kill all of the antigens in the body to clear the virus. B cells allow you to gain immunity from a particular disease because they stick around in the body for a long time in the form of “memory” B cells. If you get the same virus later on, these memory B cells will reactivate and create antibodies to kill the virus.
Ch. 13: The Discerning Cell
- T Cells: Killers & Helpers — T cells are white blood cells that operate in the “adaptive immune system” alongside B cells and antibodies. Surprisingly, T cells were only discovered about 50 years ago (in the 1970s). They are produced in the bone marrow, then migrate to and reside in the thymus, a bulky and soft gland that sits above the heart. There are two general types of T cells:
- Killer T Cells — Killer T cells take care of the problem when a virus or bacterium has snuck inside a cell and infected it. They constantly survey cells in the body and have a special ability to detect cells that have been compromised by a virus. They then kill those infected cells, effectively killing the virus inside.
- Helper T Cells — Helper T cells help deal with viruses or bacterium that have been detected outside of a cell. They essentially detect when a virus has first entered the body (before it has gone inside of our cells) and orchestrate a response by telling B cells to make antibodies and summoning other immune system cells to the scene. Helper T cells act like a point guard, telling different players in the immune system what to do to challenge the infection before it gets inside our cells.
- “Killer” T Cells: How They Work — One of the problems with a virus or bad microbe is that they can get inside a cell and turn the cell against itself. Antibodies produced by B cells (discussed in the previous chapter) cannot get inside of cells, so they are of little help in these scenarios. So who solves this problem? Killer T cells do. T cells are able to discern a virus-infected cell from an uninfected cell, and then kill the virus-infected cell. In short, killer T cells are known for their ability to tell which cells are your cells, and which cells have gone bad (due to a virus or bacteria). They then kill the cells that have turned against you and become sort of the “nonself.” These T cells are killers. . . assassins.
- Quote (P. 207): “But what if a virus has taken residence within a cell? What about, say, a flu virus that has infiltrated the cell and hijacked its protein-making apparatus in order to churn out viral proteins that are indistinguishable from the cell’s own? This is what viruses do: they ‘go native.’ A flu virus turns its hostage into a veritable flu factory, producing thousands of virions per hour. And because antibodies cannot enter cells, how are they to identify one of these rogue cells masquerading as a normal cell? What, then, prevents any virus from using every cell in our body as a perfect microbial sanctuary? The answers to all these questions, I would soon find out, lay in the cell whose seductive song had tugged me all the way from California to Alain Townsend’s lab at Oxford; the cell that could, with nearly miraculous sensitivity, discern a virus-infected cell from an uninfected one, and the cell that can discriminate the self from the nonself. The subtle, wise, discerning T cell.”
- T Cells: Two Conditions to Kill — Killer T cells are constantly monitoring the cells in the body. They’re like military captains closely watching every soldier in line. There are two conditions that would lead to a T cell making a kill. If both of these conditions are met, the T cell will make the kill. These two conditions are:
- Self vs. Nonself — Does the cell in question belong to the body? In other words, if it’s a cell from an outside body (e.g. you’re trying to implant a stranger’s cells into your body), the T cell will make the kill because it’s considered “nonself.” This is why many grafts are rejected.
- Infected vs. Not Infected — Second, is the cell in question infected with a virus or bacterium? If it is, the T cell makes the kill. They have a knack for being able to tell when a virus has moved inside of a cell and infected it. Other immune system players (e.g. antibodies) have a hard time telling. In these situations, T cells make the kill.
- Quote (P. 208): “A second feature of killer Ts was just as puzzling. Although a CD8 T cell could recognize a cell from the same body, it killed only infected cells from the same body. No viral infection, no kill. It was as if the T cell was capable of asking two independent questions. First: Does the cell that I am surveying belong to my body? In other words, is it self? And second: Is it infected with a virus or a bacterium? Has the self been changed? Only if both were true — the self and infection — would a T cell kill its target.”
- “Helper” T Cells: How They Work — Helper T cells are primarily involved when a virus or bacterium is outside of our cells and has not snuck inside yet. They essentially bridge the gap between the “innate immune system” and the “adaptive immune system.” They do this by detecting when neutrophils and macrophages of the innate immune system have started battle with a virus or bacterium. Having detected the invader, they begin to coordinate an immune response by telling B cells to make antibodies and summoning other immune cells to the scene. They act like a point guard, telling different cells what to do to challenge the invader. These are critical to a healthy immune system.
- Quote (P. 215): “In the absence of the CD4 cell [Helper T Cell], the transition between innate immunity and adaptive immunity — i.e., between the detection of a pathogen and antibody production by B cells — would fall apart. For all these properties, and especially for supporting the B cell antibody response, this type of cell is called the ‘helper’ T cell. Its job is to bridge the innate and adaptive immune system —macrophages and monocytes on one end, and B and T cells on the other.”
- Quote (P. 219): “The CD4 cell [helper T cell] is not so much a helper as it is the master machinator of the entire immune system, the coordinator, the central nexus through which virtually all immune information flows. . . It [helper T cell] is the central bridge between innate immunity and adaptive immunity — between all the cells of the immune system. The collapse of CD4 cells [helper T cells] thus cascades rapidly into a collapse of the immune system in total.”
- T Cells vs. B Cells: Speed of Operation — One of the big differences between T cells and B cells, other than their primary functions, is the speed at which they work. T cells generally work far slower than B cells. B cells react to an invader almost immediately; creating antibodies that can be released into the blood to go fight the virus’s antigens. T cells work much slower; they are almost like Sherlock Holmes solving a detective case. T cells constantly survey our cells, looking for any trace of infection. When they find it, they eliminate the bad cell. B cells and antibodies are far quicker. But it’s this duality in speed that allows us to get rid of a virus in full; B cells and antibodies clear the antigens in the blood, while T cells slowly and methodically eliminate cells that the virus has climbed inside and infected.
- Quote (P. 216): “Antigen processing and presentation to CD4 [helper T cells] and CD8 [killer T cells] cells — the mainstays of T cell recognition — are slow but painstakingly methodical processes. Unlike an antibody, a gunslinging sheriff itching for a showdown with a gang of molecular criminals in the center of town, a T cell is the gumshoe detective going door to door to look for perpetrators hiding inside.”
- Quote (P. 216): “Unlike the B cell, though, the T cell isn’t looking for a culprit to come bursting out of the saloon, guns a-blazing. It is, like some omniscient Sherlock Holmes with a pipe and an umbrella, seeking the portent of a person.”
- Quote (P. 216): “It’s this duality that ensures that viruses and bacteria are not just cleared from the blood by antibodies but are also cleared from infected cells where they could otherwise be harbored safely, by T cells.”
- AIDS: Low Helper T Cell Count — AIDS burst onto the scene in the late 1979s and early 1980s. At first, the disease was a complete mystery. But as scientists looked into the cellular behavior of some of the first AIDS patients, they found the problem: the patients exhibited helper T cells (CD4 cells) that were dysfunctional and very low in overall count. These patients had CD4 counts in the 50s when a normal count is in the 500-1,500 range. The patients’ immune systems were failing because of a very low helper T cell count. Helper T cells are the master orchestrators of the immune system; low counts are devastating to the body.
- Quote (P. 218): “By sifting through each type of immune cell, the crucial defect was soon identified as a dysfunctional CD4 helper T cell that was also low in number. A normal CD4 count is around 500 to 1,500 cells per cubic millimeter of blood. Patients with full-blown AIDS had only 50, or even 10. AIDS, as one group of researchers described it, was ‘the first human disease to be characterized by the selective loss of a specific T cell subset, namely, CD4+ T helper/inducer cells.’”
- AIDS & HIV — It didn’t take long for scientists to come to the conclusion that some kind of virus was causing low helper T cell counts in AIDS patients. The virus was eventually called human immunodeficiency virus (HIV). The virus could be transmitted sexually, during blood transfusion, and from infected needles introduced into the blood stream, usually through intravenous drug use.
- Chapter Takeaway — T cells are white blood cells that are part of the “adaptive immune system.” T cells generally fall into one of two categories: killer T cells and helper T cells. Killer T cells act like a military commander, constantly surveying all cells in the body, looking for invaders. They can discern between healthy and infected cells. When they find a cell that has been infected by a virus or bacterium, they kill it. Helper T cells are master orchestrators of the immune system: they help lead a coordinated response to an invader by telling B cells to make antibodies, summoning other cells from the immune system to the scene, and more.
Ch. 14: The Tolerant Cell
- T Cells: Knowing the Self — As noted in the previous chapter, one of the amazing aspects of T cells, specifically killer T cells, is that they are able to tell which cells are part of your body and which aren’t at all (another person’s cell) or are altered (our own cells infected by a virus). T cells are why many grafts do not work; your T cells recognize that the cells in question are somebody else’s cells and reject them.
- T Cells: Recognizing Self vs. Nonself — The way T cells are able to tell if a cell is somebody else’s involves genes called ‘H genes’. In short, every cell in your body has H2 proteins that are different from the proteins in a stranger’s cells. When a stranger’s cells are inserted into or onto your body, T cells recognize these H2 proteins as different and reject them.
- Quote (P. 231): “He termed them H genes, for histocompatibility genes —histo from tissue, and compatibility because of their capacity to render foreign tissue to be accepted as self. It was some version of these H genes, Snell realized, that defines the boundary of the immunological self. If organisms shared the H genes, you could transplant tissue from one organism to another. If they didn’t, the transplant would be rejected.”
- Quote (P. 231): “How does the self know itself? Because every cell in your body expresses a set of histocompatibility (H2) proteins that are different from the proteins expressed by a stranger’s cells. When a stranger’s skin, or bone marrow, is implanted into your body, your T cells recognize these MHC proteins as foreign — nonself — and reject the invading cells.”
- T Cells: Recognizing the “Altered Self” — T cells are also able to distinguish altered cells from normal cells. Altered cells are ones in which a virus or bacterium has entered inside and infected. Once again H2 proteins are involved in helping T cells detect these compromised cells. In this case, these proteins have a way of turning the cell “inside out” so it shows its true colors. T cells then see that there has been an infection and kill the compromised cell.
- T Cells: Staying In Line — What prevents T cells from just destroying all cells (self and nonself)? There are a couple things that happen, as described below. T cells are born in the bone marrow before migrating to the thymus (located just above the heart) to live and mature. The process of keeping T cells in check happens both inside and outside the thymus. There are also “checkpoints” on the surface of T cells that act like safety locks on a gun and prevent T cells from firing on normal cells.
- Inside the Thymus — Scientists have found that something happens in the thymus where T cells that show signs of rebelling against normal cells are “deleted” from the immune system before they make it out of the thymus. This process is called “central tolerance.”
- Quote (P. 234): “But, much as Burnet had predicted, they found that the immature T cells that recognized pieces of that protein — the ones that attacked the self — were deleted in the thymus by way of a process called negative selection.”
- Outside the Thymus — Central tolerance isn’t enough to guarantee that T cells won’t end up attacking the self. When T cells leave the thymus, they have to be held in line. Cells called ‘T Reg’ cells help with this by acting as “T cell policemen.” Essentially, they keep T cells in check, acting like policemen to make sure T cells behave and don’t start attacking the self (normal cells). When scientists have removed ‘T Reg’ cells, the results have been devastating: an autoimmune disorder develops as T cells go crazy and attack the body’s normal cells.
- Checkpoints — In the 1970s, scientists discovered yet another mechanism that helps prevent T cells from attacking normal cells. The mechanism involves proteins on the T cell’s surface that have to be activated before a T cell attacks. These proteins act as checkpoints. Think of safety switches on a gun. An invader has to meet the right qualifications to activate all of the checkpoints. These checkpoints prevent T cells from firing on normal cells.
- Quote (P. 237): “These backups beyond backups — mountains beyond mountains — are like trigger locks and safety switches in guns, and they had evolved to ensure that T cells did not accidentally direct their friendly fire on normal cells. The trigger locks would act as checkpoints against the indiscriminate killing of our own cells.”
- Quote (P. 240): “Over time, researchers have discovered that T cells have multiple checkpoints. Each acts as a safety switch to prevent trigger-happy T cells from attacking the self.”
- Inside the Thymus — Scientists have found that something happens in the thymus where T cells that show signs of rebelling against normal cells are “deleted” from the immune system before they make it out of the thymus. This process is called “central tolerance.”
- Autoimmune Disorders — Autoimmune disorders occur when T cells attack your normal cells. In other words, when T cells attack you. The checks and balances mentioned above do a great job of making sure T cells stay focused on killing infected cells and cells from other people, but there are times where these guardrails fail and T cells begin killing your normal, healthy cells. Friendly fire. There are so many different kinds of autoimmune disorders, and all of them are very scary. It’s literally your body turning against you.
- Cancer — Cancer cells are very difficult to figure out. They are extremely smart and resourceful, which is why we still struggle to beat them. There are so many different kinds of cancer, but they all involve your cells turning against you. What makes them so tricky is that they are invisible to our immune system. They look like normal cells to all of our immune system players. As a result, they are able to go about their business of killing you, undetected, and without facing a lot of opposition. Once a cancer has spread enough, there’s nothing the body can do; the cancer cells have overtaken it. This is why it’s so important to detect cancer early. There are many things that make cancer cells so hard to overcome. Below are three big ones:
- Rogue Self — Cancer cells appear like normal cells (the self). They produce the same proteins as normal cells. They act like normal cells. The difference is that they have evil intentions; rather than serving you (like normal cells), they have one objective: kill you. They use all of their powers against you.
- Quote (P. 238): “Harold Varmus, the Nobel-winning cancer biologist, called cancer a ‘distorted version of our normal selves.’ And so they are: the proteins that cancer cells make are, with a few exceptions, the same ones made by normal cells, except cancer cells distort the function of these proteins and hijack the cells toward malignant growth. Cancer, in short, may be a rogue self — but it is, indubitably, a self.”
- Invisibility — Because they look and act like normal cells, cancer cells go undetected by the immune system. They are able to do their damage without much of a fight, because our T cells and other cells in the immune system simply don’t recognize them as invaders with bad intentions.
- Quote (P. 238): “Cancers, varied as they are, share some common features — among them, their invisibility to the immune system.”
- Building Barriers — Cancer cells are able to surround themselves with normal cells that are resistant to drugs, in effect creating a barrier of protection
- Quote (P. 238): “Cancer cells evolve to create unique cellular environments around themselves — typically by surrounding themselves with normal cells — that cannot be penetrated by drugs, or that can actively cause drug resistance.”
- Quote (P. 238): “One of the most terrifying scientific images that I have seen in recent times is a tumor surrounded by a shell of normal cells that has excluded activated T cells. The T cells form a ring around the cellular carapace that the cancer has formed around itself, but are unable to penetrate it.”
- Rogue Self — Cancer cells appear like normal cells (the self). They produce the same proteins as normal cells. They act like normal cells. The difference is that they have evil intentions; rather than serving you (like normal cells), they have one objective: kill you. They use all of their powers against you.
- Cancer: Chemo vs. Immunotherapy — There are a number of ways to try to treat cancer. The most well-known way involves chemotherapy, where the goal is to attack all cells in the area in an effort to kill the cancer cells. Unfortunately, this results in horrible side effects and baldness because the treatment is killing normal, healthy cells. There’s no way to target cancer cells exclusively with chemo. Immunotherapy is becoming more and more viable. There are several ways to execute immunotherapy, but the general idea is to shine a light on the cancer cells so they are no longer invisible to the immune system. There are also techniques that involve withdrawing T cells, arming them with weapons (so to speak) then reinserting them back into the body to try to beef up the attack. Below are a few types of immunotherapies:
- CAR-T — Interestingly, CAR-T treatment involves removing T cells (the immune system’s assassins) from a patient’s blood, loading them up with weapons to fight cancer cells by using genetic engineering to add antigen receptors specifically targeted to the patient’s tumors, and then infusing them back into the patient. The modified T cells then go in and wipe out the patient’s cancer. Unfortunately, this treatment has only been proven to be effective against B-cell lymphoma.
- Checkpoint Inhibitors — These drugs take the opposite approach by making cancer cells visible to the immune system. In other words, they “shine the light” on cancer cells, exposing them to normal T cells, which go in and kill the bad cancer cells. The drugs do this by blocking the “checkpoints” that are in place to prevent T cells killing normal cells. With these checkpoints blocked, T cells are more likely to fire on cancer cells instead of floating right by them. The downside is that they might start firing on normal cells as well. One of the men who developed checkpoint inhibitors, James Allison, won a Nobel Prize for his work in 2018. These drugs also helped save the life of former President Jimmy Carter a few years ago.
- ACT — ACT is a class of immunotherapy whereby supplemental T cells are transferred into a patient, like adding reinforcements to an army, to bolster their ability to fight their own tumor. These T cells have been genetically programmed with antigens specifically targeted at the patient’s individual tumor type. It is similar to CAR-T cell therapy, but much broader in scope. As cancer grows, it quickly outruns the immune system’s ability to detect and kill it; there simply aren’t enough T cells to do the job. ACT attempts to overwhelm the cancer by sending in an army of targeted T cells.
- Blood: A Summary — As this chapter wrapped up, Mukherjee beautifully summarized the various cells that make up the blood. Each have been discussed in the previous few chapters. The cells of the blood and immune system truly resemble a civilization, each cell carrying out specific tasks to keep the body healthy. It’s really miraculous. The quote below is Mukherjee’s summarization:
- Quote (P. 242): “Blood. A cosmos of cells. The restless ones: red blood cells. The guardians: multilobed neutrophils that mount the first phases of the immune response. The healers: tiny platelets — once-dismissed as fragmentary nonsense — that redefined how we respond to breaches in the body. The defenders, the discerners: B cells that make antibody missiles; T cells, door-to-door wanderers that can detect even the whiff of an invader, including, possibly, cancer.”
- Chapter Takeaway — T cells have a remarkable ability to tell self from nonself as they survey the body’s cells, and there are multiple measures in place to prevent T cells from opening fire on normal cells (the self). When T cells do escape all of the checks and balances and misfire on normal cells, it becomes an autoimmune disorder. These are really scary.
Ch. 15: The Pandemic
- SARS-COV2: Coronavirus — Coronavirus (SARS-COV2), also known as COVID-19, swept the globe in 2020. By most reports, the virus originated in China and was first experienced in the U.S. in Washington in January 2020. It ultimately infected over 500 million people and killed more than 6 million. The virus took years to fully defeat — there were at least three different strains that allowed the virus to linger on.
- Coronavirus & Cell Biology — There were three main features of the coronavirus that caused it to escalate into a full-blown global pandemic. These factors made it difficult to fully tame the virus, which is why it lingered on for so long. These include:
- Asymptomatic — Many people who had COVID often didn’t know they had it because they didn’t feel symptoms until at least a few days after they had been infected with it. This made things very difficult, especially in the beginning of the pandemic. Many people were going about their daily lives with no awareness that they were infected. They were asymptomatic.
- Exponential Growth — The “contagiousness” of the virus was at least three, meaning one person can infect at least three others. This feature of the virus allowed it to spread like wildfire.
- Mysterious & Deadly — COVID was deadly; millions of people died from this virus. The primary symptom that caused death was not being able to breathe properly; COVID had a major impact on the respiratory system. At the same time, mysteriously, others infected with COVID only experience mild symptoms.
- COVID: How It Worked — Some people died from COVID. Others only experienced mild symptoms. Why was this the case? There were a few things going on here that caused so many issues for the body. These include:
- TLR7 Sensor — Scientists found that one of the main issues had something to do with the TLR7 sensor built into every cell. This sensor “lights up” when an infection is first recognized in the body. When it lights up, the immune system ramps up to fight, beginning with the players in the innate “immune system.” The problem? Some people have a TLR7 sensor that isn’t working right, which means the body was never alerted to the fact that COVID had come in and infected it. In short, the immune system didn’t respond to the virus right away. The people with dysfunctional TLR7 sensors were the ones that generally had the worst experience with COVID. People who had normal TLR7 sensors generally didn’t have too much of a problem with COVID because their system detected the virus and took care of it.
- Carpet Bombing — Eventually, infected cells were finally able to pull a second alarm to signal danger (cytokines). This led to the immune system sending a huge number of soldiers (immune cells) to take down the virus. These soldiers carpet bombed the virus, relaxing a lot of toxins to kill the infected cells. This caused a ton of fluid from dead cells. The fluid clogged the air sacs, making it difficult to breathe. People who got the worst of COVID were experiencing oxygen levels in the 30-50% range.
- Quote (P. 253): “The war against the virus — as much as the virus itself — became an escalating crisis. The lungs were bogged with fluid; debris from dead cells clogged the air sacs. ‘There appears to be a fork in the road to immunity to Covid-19 that determines disease outcome,’ Iwasaki told me. ‘If you mount a robust innate immune response during the early phase of infection [presumably via an intact TLR7], you control the virus and have a mild disease. If you don’t, you have uncontrolled virus replication in the lung that fuels the fire of inflammation leading to severe disease.’”
- Chapter Takeaway — Coronavirus led to one of the deadliest global pandemics of all time. People who had faulty TLR7 sensors in their cells had the most difficult time with COVID; their sensors were not going off to alert the immune system of an infection. When they were finally able to alert the immune system, they got carpet bombed by the huge number of immune cells sent in to attack, causing fluid in the air sacs from dead cells. People with normal TLR7 sensors in their cells generally only experienced mild symptoms.
Ch. 16: The Citizen Cell
- Organs — An organ is a unit in the body in which cells come together to serve a common purpose. Cells in organs carry out general cell tasks already discussed in the book (e.g. protein synthesis, metabolism, waste disposal, autonomy), but they are also specialists: they carry out unique functions that serve that specific organ that they represent. Together, these specialized cells in organs are able to generate things like a heartbeat (heart cells) and a thought (brain cells).
- The Heart — The heart will beat more than 2 billion times over an average person’s life. Through its contracting and relaxing actions, the heart pumps blood to the body, using veins and arteries as channels of transportation. The heart is made up of two pumps — one left and one right, laid side by side, like twins in a womb. It’s all a circle. Below is how it works:
- Right Pump — Everything starts with the right side. The right-sided pump collects blood from the veins of the body. Exhausted and depleted after completing a circuit around the body, incoming blood from the veins (which is a darker shade of red than usual) pours into the upper right chamber called the right atrium. It then passes through a valve and is moved into the pumping chamber, the right ventricle. A powerful heave from the right ventricle pumps the blood to the lungs. This is the right-sided circuit — veins to heart to lung.
- Left Pump — The lungs, having received blood from the right side of the heart, oxygenate the blood and clear the carbon dioxide. Now clean and fueled with oxygen, blood, now a slightly lighter shade of red (crimson red), moves to the left side. It collects in the left atrium of the heart. It is then pushed into the left ventricle. It is this left ventricle, perhaps the most tireless muscle in the body, that ejects the blood forcefully into the wide arc of the aorta, the major blood vessel that carries oxygenated blood to the body, and to the brain. Round and round in circles.
- Heart Cells: Actin & Myosin — There are two fibers found in all muscle cells in the body: actin and myosin. These fibers in heart cells are responsible for the contract-relax motion that the heart performs. They slide against each other to help the muscle contract. This process requires a lot of energy, which is why every heart cell and muscle cell in the body has a lot of mitochondria to supply the energy needed for the two fibers to slide. More on actin and myosin’s role in contracting muscles:
- Quote (P. 267): “The trick to a muscle cell’s contraction is that these two fibers — actin and myosin — slide against each other, like two networks of ropes. When a cell is stimulated to contract, a part of the myosin fiber binds to a site on the actin fiber, like a hand from one rope gasping the other. It then un-clutches it and reaches forward to bind to the next site — a man suspended on one rope, grasping and pulling on the other, one fist upon the next. Clutch. Pull. Release. Clutch. Pull. Release. Each muscle cell has thousands of such lined ropes — bands of actin in parallel with bands of myosin. As the ropes, lined side by side, slide against each other — clutch, pull, release — the edges of the cell are also yanked, and the cell is dragged into a contraction. The process requires energy, of course, and every heart cell and muscle cell is chock-full of mitochondria to supply the energy required for the two fibers to slide.”
- Chapter Takeaway — For the heart to function properly, all of its cells must contract in a coordinated, harmonious order. The heart’s job is to pump blood around the body. The heart is made up of two pumps and uses arteries and veins as highways to send blood throughout the body.
Ch. 17: The Contemplating Cell
- Neurons — Neurons are considered the most essential cells of the brain. Together, the brain’s neurons make up the nervous system. The nervous system is a network of wires and circuits in which messages are sent via neuron-to-neuron impulses. Unlike the heart, where all of the organ’s cells are in perfect sync and march together, neurons power the brain by connecting and talking to each other in a “wired” type of setup. They fire off of each other. It’s like a chain reaction. Structurally, individual neurons consist of many different shapes and sizes. At their core, however, they share many common features. These include:
- Cell Body (Soma) — All neurons have a cell body
- Dendrites — Sprouting from the cell body are dozens, hundreds, or even thousands of branch-like things called dendrites
- Axon — An axon is the neuron’s outflow tract that extends out toward the next cell. This is the neuron’s outflow point
- Synapse — In between one cell’s extended axon and the next neuron is a small space called a synapse. In this way, the nervous system is wired but the “wires” consist of cells connected to cells connected to cells, with intervening spaces (synapses) between them.
- Nervous System: How It Works — Information moves through the brain and nervous system via electrical and chemical impulses from cell to cell. How does this work? Information travels unidirectionally in a nerve. The dendrites — those extensions budding out of the neuron’s cell body — “receive” the impulse. The impulse then moves through the cell body. It then moves out through the axon, through the synapse, to the next nerve cell. The process is then repeated in the next cell; its dendrites pick up the impulse, transmit it to the cell body, and then the impulse flows out through the axon to the next cell. This process repeats forever. Mukherjee summarizes the process in more detail below:
- Quote (P. 287): “Neurobiologists studying the synapse had largely described the communication between neurons to be a rapid process. An electrical impulse arrives at the end of the neuron — i.e., at the axon terminal. It causes the release of chemical neurotransmitters into a specialized space — the synapse. The chemicals, in turn, open channels in the next neuron, and ions surge in, reinitiating the impulse. This is the ‘electrical’ brain — a box of wires and circuits (with a chemical signal — a neurotransmitter — thrown in between the two wires).”
- Impulses: Electrical & Chemical — Information is transferred from cell to cell, nerve to nerve, and nerve to muscle in endless cycles via electrical and chemical impulses in the brain. Both types are needed to make the brain’s neural wiring and circuiting work. Why? Think of a laptop. A laptop’s internal wiring is all electrical wiring; it can help you do work, but it can’t read your mental and emotional state. That’s where chemical impulses (neurotransmitters) come in. In the brain, chemical neurotransmitters respond to your mental and emotional state; tell it you are in a bad mood and it can give you feedback to stop sending out angry emails (sticking with the laptop analogy). A collection of these wired modules and circuits make up the machine that we call our brain. The quote above contains a better breakdown of this.
- Depression: Role of Serotonin — From a medical perspective, depression is the result of an imbalance of neurotransmitters (chemicals) in the brain, which leads to dysfunctional neurons (brain cells). As far back as the 1950s, many drugs created to treat depression focus on increasing the levels of serotonin in the brain — serotonin is one of the neurotransmitters in the brain, and low levels of it is linked to depression. But the problem is more complex than simply increasing levels of serotonin; drugs that have accomplished this have had mixed results. Why the mixed results? It’s more about what serotonin, or lack-thereof, does to neurons in the brain. It’s about how serotonin changes a neuron’s chemistry and metabolism. And that varies from person to person — genetics often play a large role here, which is why these drugs are effective in some people and not as much in others.
- Quote (P. 291): “Depression is a flaw in love. But more fundamentally, perhaps, it is also a flaw in how neurons respond-slowly — to neurotransmitters. It is not just a wiring problem, Greengard believes, but rather a cellular disorder — of a signal, instigated by neurotransmitters, that somehow malfunctions and creates a dysfunctional state in a neuron. It is a flaw in our cells that becomes a flaw in love.”
- Depression: Deep Brain Stimulation — Scientists are currently working on a way to alleviate depression that involves stimulating certain parts of the brain using implanted electrodes. The idea is that by stimulating the parts and neurons of the brain that are most associated with emotion, and happy/sad states, the patient will sort of reset and break free of the depression they are experiencing. If this were to succeed, you’d have people walking around hitting a button to activate the vibrator inside their skull.
- Quote (P. 296): “The point is this: the electrical stimulation of cellular circuits is trying to become a new kind of medicine. Some of these attempts might succeed; some might fail. But if these attempts garner even a measure of success, they will generate a new kind of person (and personhood) —humans implanted with ‘brain pacemakers’ to modulate their cellular circuitry. They will presumably wander about the world with rechargeable batteries in fanny packs and move through airport security saying: ‘I have a battery in my body with an electrode through my skull that sends impulses into the cells of my brain to regulate my mood.’”
- Chapter Takeaway — The brain is made up of many different wired networks. Information is relayed through the brain and nervous system via electrical impulses that pass between each neuron. Neurotransmitters (which are chemicals like serotonin) help facilitate these impulses via each neuron’s synapse, the small space between each cell. This is a lot different than the heart, where all cells move together in complete unison to create, for example, a heartbeat.
Ch. 18: The Orchestrating Cell
- Hormones — To this point, most of the cells discussed in this book, other than the cells of the immune system, have been local cells (e.g. heart cells, brain cells). But how does the body communicate as a whole? How do the various organs and cells of the body talk to each other, especially when there’s a problem that affects the entire body, like starvation, stress, or diabetes? The answer is hormones. Hormones act as signals that move between cells, informing them of the global “state” that the body is in. They move from one organ to the next, carried by blood. An example of a hormone is insulin or cortisol.
- Quote (P. 297): “Some signal, or impulse, must move between cells, informing them of the global ‘state’ that the body is inhabiting. The signals move from one organ to the next, carried by blood. There must be a means for one part of the body to ‘meet’ a distant part of a body. We call these signals ‘hormones’ . . . In a sense, they impel the body to act as a whole.”
- The Pancreas — The pancreas is a leaf-shaped organ (made up of pancreatic cells) tucked in near the abdomen, between the stomach and the intestines. At first, centuries ago, the gland was thought of simply as a cushion for the stomach, a support system to keep the stomach from crushing the blood vessels against the spine. Physiologists later discovered a large “duct” inside the pancreas that heads out toward the intestines. The duct acts as a channel that carries substances out to the body, such as the two below. These two substances are secreted by the pancreas (pancreas cells):
- Digestive Juices — By creating “acinar cells,” the pancreas secretes (creates) digestive juices that enable digestion by breaking down fats, starches, and protein. These digestive juices break food down into small particles, in essence converting complex food molecules into simpler, digestible units. The pancreas’s duct is the central channel for these juices.
- Insulin — Mixed in with acinar cells within the pancreas are clusters/islands of cells called islets. Islets create insulin. In this way, via islet cells, the pancreas secretes insulin, a hormone that helps regulate blood sugar levels in the blood. Insulin senses and regulates glucose (sugar) in the blood. Dysfunction of pancreatic cells, especially islet cells, is what leads to diabetes, which is characterized as very high blood glucose levels resulting from the body’s inability to clear sugar from the blood.
- Diabetes & Insulin Discovery — Diabetes occurs when the body cannot adequately sense, and lower, blood glucose levels in the blood when they get elevated. When excess glucose (via sugar or carbs) builds up in the blood, insulin is released from the pancreas to help clear it. When there is dysfunction somewhere in that process — whether it’s via insulin resistance in cells or problems with islet cells in the pancreas — it can lead to diabetes. Prior to insulin’s discovery in the early 1920s by Frederick Banting and his team, diabetes was a death sentence. It was a horrific, horrific disease. Banting won the Nobel Prize for the discovery of insulin in 1923.
- Quote (P. 302): “A disease of sugar metabolism — when the body cannot sense or adequately signal the presence of sugar, causing sugar to build up in blood and spill out in urine — diabetes was a mystifying illness.”
- Quote (P. 302): “But what if the islet cells secreted a second chemical — an internal substance — that sensed and regulated glucose? The dysfunction of these cells would make the body unable to sense glucose and send the levels of the sugar spiking sky-high in the blood — the fundamental hallmark of diabetes.”
- Quote (P. 306): “Soon, it was evident that insulin was the master regulator of sugar metabolism — the hormone responsible for sensing sugar and sending a signal to the cells throughout the body.”
- Insulin: How It Works — Insulin is created by islet cells in the pancreas and secreted into the blood to perform two key functions: to sense and clear excess glucose (from sugar and carbs). More on these two crucial functions of insulin below:
- Sensing Glucose — When insulin production is normal, the hormone helps the body sense that glucose (from sugar or carbs) is in the blood. From there, it can use the glucose for energy or clear it if there is too much of it. But if insulin is not being created by the pancreas, the body has no idea that glucose is in the blood. This is key because glucose is a huge source of energy for our body. Without the knowledge that glucose is available in the blood, the body begins to turn to fat and muscle stores for energy, which is why people with Type 1 diabetes used to be so rail thin. Type 1 diabetes occurs when the pancreas literally cannot sense the presence of sugar in the blood because its immune system has attacked the islet cells that create insulin. No insulin, no sense that there is glucose in the blood.
- Clearing Glucose — Insulin “clears” excess glucose (from sugar and carbs) in the body by moving it from the blood into cells for one of two uses: storage or energy use. Type 2 diabetes usually occurs when cells are resistant to insulin, meaning they don’t accept as much of the excess blood glucose as they should, meaning blood glucose levels remain high.
- Type 1 and Type 2 Diabetes — Building on the bullet above, diabetes comes in two forms. Both essentially come from chronically high blood sugar levels in the body. Although it is no longer a death sentence like it used to be, diabetes is a growing problem — between 1980 and 2014, the number of adults with diabetes went from 100 million to over 400 million. This trend is primarily the result of our poor eating and exercise habits. The two types of diabetes are:
- Type 1 Diabetes — With Type 1 diabetes, the body just stops producing insulin altogether. Insulin is the hormone released by the pancreas to help get rid of excess sugar in the bloodstream. Why would the pancreas not produce insulin? In the case of most Type 1 diabetes patients, their immune system attacks the islet cells in the pancreas that make insulin. As a result, the body can’t sense the presence of sugar. This means the body begins to use other sources for energy, like fat and muscle. This is why, prior to the discovery of insulin, diabetes was a death sentence: people were rail thin because the body was using fat and muscle storages for entry rather than glucose. More on how this works below:
- Quote (P. 306): “Type 1 diabetes, which affects several million patients around the globe, is a disease in which immune cells attack the beta-islet cells of the pancreas. Without insulin, the body cannot sense the presence of sugar — even if there is enough of the chemical in the blood. The cells in the body, imagining that the body has no sugar, begin to scramble around for other forms of fuel. The sugar, meanwhile, all readied up but with nowhere to go, spikes threateningly in the blood, and spills into the urine. Sugar, sugar everywhere — but not a molecule in cells to satiate them. It is one of the defining metabolic crises of the human body — cellular starvation in the presence of plenty.”
- Type 2 Diabetes — With Type 2 diabetes, insulin is produced by the pancreas, but it is less effective in the body. This either means (a) the pancreas isn’t producing enough insulin to dispatch the excess sugar in the bloodstream; or (b) the cells in your muscles, fat, and liver are insulin resistant, meaning they don’t accept the insulin your body is producing, so your blood sugar levels stay elevated.
- Type 1 Diabetes — With Type 1 diabetes, the body just stops producing insulin altogether. Insulin is the hormone released by the pancreas to help get rid of excess sugar in the bloodstream. Why would the pancreas not produce insulin? In the case of most Type 1 diabetes patients, their immune system attacks the islet cells in the pancreas that make insulin. As a result, the body can’t sense the presence of sugar. This means the body begins to use other sources for energy, like fat and muscle. This is why, prior to the discovery of insulin, diabetes was a death sentence: people were rail thin because the body was using fat and muscle storages for entry rather than glucose. More on how this works below:
- Maintaining Homeostasis: The Big Four — The pancreas, kidneys, brain, and liver are critical organs, particularly when it comes to maintaining equilibrium and homeostasis in the body. When we eat a huge meal, for example, the big inflow of carbs, nutrients, alcohol, and salt from the food needs to be metabolized and facilitated in order to return our body back to equilibrium. These four organs play a major role in that complex process. Below is a little more on how these organs operate, using an example of somebody who just ate a big meal:
- Pancreas — As mentioned already in this chapter, the pancreas is what keeps our blood glucose levels stable via the secretion of insulin. When the sugar and carbs from the meal come rushing in, the pancreas secretes insulin to recognize the glucose and move it to our cells for storage or energy use, thereby bringing our blood glucose level back down to a normal level.
- Kidneys — The huge inflow of salt (sodium) from the meal is handled by the kidneys. If the kidneys didn’t do their thing, our blood would become ocean water. The kidneys regulate certain hormones that help get excess salt out of the body. Two of the ways sodium levels are brought back to a normal level include:
- Pee It Out — Essentially, the sodium from the meal circulates in our blood until it makes its way to the kidneys, which have something called a nephron (a mini-kidney) that filters out the sodium and other waste products and helps move them into the bladder, where they can be peed out, restoring equilibrium. Stuff that can be used by the body is retained by the filter, but excess sodium and waste are not and are peed out.
- Retain More Water — The salt is also detected by specialized cells in the brain that monitor the overall concentration of salts in the blood, a system called osmolality. These cells, sensing a high osmolality, send out another hormone to cause the cells in the kidney to retain more water. As more water is absorbed into the body, the sodium level in the blood is diluted, and the concentration is reset — albeit at the cost of retaining more water overall. This is why we normally weigh more the next day after eating high-sodium meals — the body is purposely retaining more water in order to dilute the high amount of sodium in the body. It is attempting to restore equilibrium.
- Liver — How is alcohol from the meal dealt with? The liver handles this part. Liver cells do a ton of different things for the body, but one involves waste disposal. Unlike the kidneys, which basically filter and send waste out of the body via the urine like a sewer system, the liver has dozens of ways of detoxifying and dispensing waste. In one system, it generates a sacrificial molecule that attaches itself to a potentially toxic one (e.g. from alcohol) and renders it inactive; both the sacrificial molecule and the toxin are then broken down further until the poison is detoxified. For other waste products, it destroys the chemical using specialized reactions. Alcohol, for instance, is detoxified in a series of reactions, until it is broken down into a harmless chemical. There are even specialized cells within the liver that eat dead or dying cells — red blood cells, for instance. Reusable products from the dead cells are recycled. Others are dispensed into the intestines or excreted by the kidney.
- Quote (P. 311): “The pancreatic cell maintains metabolic constancy, the kidneys salt constancy. The liver maintains chemical constancy.”
- Quote (P. 312): “The liver, pancreas, brain, and kidney are four of the principal organs of homeostasis. The pancreatic cells control metabolic homeostasis through the hormone insulin. The kidneys’ nephrons control salt and water, maintaining a constant level of salinity in the blood. The liver, among many of its functions, prevents us from being soused in toxic products, including ethanol [alcohol]. The brain coordinates this activity by sensing levels, sending out hormones, and acting as a master orchestrator of balance-restoration.”
- Chapter Takeaway — No matter what, the body tries to restore itself back to equilibrium (homeostasis). The four organs that are a major part of that process are the pancreas, kidneys, liver, and brain. These organs regulate and produce various hormones (e.g. insulin from the pancreas) in an attempt to keep the body in a normalized state. If the cells of these organs are dysfunctional and have a hard time getting the body back into a normal state (after eating, for example), disease usually ensues. An example of this is Type 2 diabetes, a disease that arises when the pancreas and the insulin it secretes cannot get blood glucose levels back to normal following an inflow of sugar and carbs.
Ch. 19: The Renewing Cell
- Hiroshima & Stem Cells — On August 6, 1945, the U.S. dropped an atomic bomb named Little Boy on the Japanese city of Hiroshima, effectively ending World War II. The bomb was dropped from a B-29 bomber. It released the energy equivalent of 15,000 kilotons of TNT — about 35,000 car bombs going off at once. About 70-80,000 people, roughly 30% of Hiroshima’s population, died instantly. Many more people died later on from radiation sickness. A third wave of people also died a few weeks later. This group included people who were exposed to low levels of radiation that essentially knocked out their bone marrow, which led to an inability to produce enough blood cells for survival. This third wave of death confirmed the presence of stem cells.
- Stem Cells — Stem cells are essentially OG cells that are clones of themselves. Because they are OG cells, they have the ability to continuously replicate the type of cell they represent. Blood cells are a good example. Red blood cells are constantly dying, but we are able to replace them because our stem cells continue to pump out fresh red blood cells. For those who were in the third wave of death after the Hiroshima bomb, their blood stem cells were killed. In short, stem cells have two core functions:
- Divide — Stem cells have to be able to divide to form any kind of cell within its structure. For example, a blood stem cell has to be able to give rise to white blood cells, red blood cells, and platelets.
- Replenish — But stem cells also need to be able to divide to replenish. This is the truest form of a stem cell. This function allows the stem cell to replenish the body by replacing cells that are dying. For example, blood stem cells need to be able to replenish all of the red blood cells that die every day.
- Quote (P. 317): “But stem cells are themselves a biological paradox. Their two principal functions seem, at face value, to be precisely opposed to each other. On one hand, a stem cell must generate functional ‘differentiated’ cells; a blood stem cell, for instance, must divide to give rise to the cells that form the mature elements of blood — white cells, red cells, platelets. But on the other hand, it must also divide to replenish itself — i.e., a stem cell. If a stem cell achieved only the former function — differentiation into mature, functional cells — the reservoir of replenishment would eventually be exhausted. Over the course of adulthood, our blood counts would keep falling year after year, until there were none left. In contrast, if it only achieved its own replenishment — a phenomenon termed ‘self-renewal’ — there would be no production of blood.”
- Quote (P. 317): “It is the acrobatic balance between self-preservation and selflessness — self-renewal and differentiation — that makes the stem cell indispensable for an organism, and thereby enables the homeostasis of tissues such as blood.”
- Stem Cells: Creating a “Backup Copy” — To try to drill home the concept of a stem cell, think of it as the OG cells of an organism creating “backup copies” of themselves. That way, if the OG cell were to die via injury or illness, the stem cell would take its place and be able to divide and replenish cells within its structure.
- Quote (P. 318): “Like most real stem cells, these mythic doubles usually lie dormant — quiescent — until an injury wakes them up. And then they awake and reseed the whole clan. It results not in birth but rebirth.”
- Stem Cells & Bone Marrow Transplants — All components of blood — red blood cells, white blood cells, and platelets — are formed in the bone marrow. Blood stem cells are also located there. In total, 95% of the body’s blood cells are made in the bone marrow. The discovery of stem cells in the mid-1900s had a major impact on how we view bone marrow. For people who are anemic and can’t produce enough blood cells to keep the body replenished via their own blood stem cells have hope with a bone marrow transplant. By using donated bone marrow from a person who has healthy stem cells, doctors can completely regenerate the blood system in a sick patient.
- Quote (P. 322): “The effect on the field of bone marrow transplantation was extraordinary. If transplanters could find this [stem] cell, they could regenerate the entire blood system.”
- Quote (P. 323): “A single stem cell can produce billions of mature red and white cells — and an entire organ system of an animal.”
- Bone Marrow Transplants & Leukemia — Leukemia is cancer of the body’s blood-forming tissues, including the bone marrow and the lymphatic system. As stem cell research developed in the 1960s, 70s, and 80s, doctors began to attempt bone marrow transplants to try to save leukemia patients. The idea is that by doing a bone marrow transplant, the patient would receive new blood stem cells that could produce a whole new system of healthy blood cells capable of replacing the old blood cells and killing the cancerous blood cells. Early on, the treatment was very risky: many patients died. The problem was that the grafted bone marrow cells from the donor would often attack the host, which killed a lot of patients receiving the treatment. But for those who survived, the fact that the donor’s cells attacked their own cells meant that the donor’s cells were also attacking the leukemia. Those who survived the treatment often came out cancer-free.
- Chapter Takeaway — Stem cells are clones of the OG cells in the body that can divide to keep the body replenished at all times. Blood stem cells are a good example. These cells constantly replace the millions of blood cells we lose every day. Bone marrow transplants attempt to take a donor’s stem cells and insert them into a patient who is anemic or has leukemia.
Ch. 20: The Repairing Cell
- Bone: It’s An Organ — There’s more than meets the eye when it comes to bone in the body. First of all, it is an organ. It grows to a point, and then knows when to stop growing. It heals itself continuously throughout adult life and repairs itself after injury. It responds with sensitivity to hormones; it potentially even synthesizes its own hormones. Bone marrow serves as the birthplace of blood, including blood stem cells. And it is involved in osteoarthritis and osteoporosis, two of the major illnesses of aging.
- Bone: What It’s Made Of — On the surface, bone looks simply like a chunk of hardened calcium, but it is actually made of multiple cells. The most familiar is cartilage, but there are two lesser known cells involved in bone. They are:
- Osteoblast (Bone Makers) — This is the cell that deposits calcium and other proteins to form a calcified matrix in layers, and then gets trapped in its own deposit to form new bone. It is the bone-making, bone-depositing cell: typically, the osteoblasts thicken and lengthen the bone. To remember this easily, pretend the letter “b” in the word stands for “bone making.”
- Osteoclast (Bone Chewers) — These are large cells with multiple nuclei that are bone-eaters. They chew away on the matrix, or punch holes in it, removing and remodeling bone, like constantly pruning gardeners. To remember this easily, pretend the letter “c” in the word stands for “bone chewing.”
- Osteoblast & Osteoclast: Maintaining Homeostasis — How does bone maintain homeostasis and equilibrium, like other organs throughout the body do? The coordinated work of osteoblast and osteoclast cells is one of the ways this occurs. Osteoblast cells make bone, and osteoclast cells chew bone away. Both are needed to maintain homeostasis in the skeletal system.
- Quote (P. 339): “The dynamic balance between osteoblasts and osteoclasts — bone makers and bone chewers — is one mechanism by which bone maintains homeostasis. Take away osteoblasts and new bone cannot be laid down. Make osteoclasts — the bone chewers — defective, and the bone turns thick — ‘stone bone,’ as early pathologists called it — tough-seeming, but hard to repair. The cavities inside contract, crowding out space for the marrow, precipitating a disease called osteopetrosis.”
- Bone: Lengthening & Repairing — It’s interesting that bone can lengthen in adolescence and repair itself in adulthood. These are two of the major functions of bones, and it turns out that there are two different cells that are big contributors to both of these systems. These are:
- OCHRE Cells — How does bone grow longer in the body, particularly in adolescence? The answer is a special population of cells called OCHRE cells that Mukherjee and his lab helped discover. These cells sit at the base of growth plates at the two ends of bones and fire off cartilage and osteoblasts that lead to the bone lengthening. These cells go away over time, which is why we spring taller as kids and stop growing as adults.
- Quote (P. 342): “How does bone grow during adolescence? Well, because a special population of cells [OCHRE cells], sitting at the growth plate at the two ends of bone, shoots off cartilage and osteoblasts that allows bone to lengthen. And why does it stop growing? Because this population diminishes over time, until early adulthood, when very few are left.”
- LR Cells — So how does bone repair itself after injury if OCHRE cells diminish over time? LR cells are part of the reason. LR cells are born in adulthood and come into play when you fracture a bone as an adult. They rush to the scene to begin rebuilding the fractured bone. They also contribute to keeping your bones nice and thick as an adult.
- Quote (P. 342): “If you fracture the long shaft of bone, somewhere in the middle, the LR cells swing into action, generating bone-making cells that repair the injured long bone.”
- Quote (P. 343): “What a mess, you might say, but it’s quite the opposite. Bone is not just an organ with a single supply of rejuvenating cells; it is a chimera of rejuvenation. It has at least two sources for two sites. There are growth-plate resident OCR (or OCHRE) cells, which form lengthening bone. They arise early in development and then gradually decay with age. And there are LR cells that arise later in adolescence and adulthood that participate in the maintenance of thickness of long bones, and bone fracture repair.”
- OCHRE Cells — How does bone grow longer in the body, particularly in adolescence? The answer is a special population of cells called OCHRE cells that Mukherjee and his lab helped discover. These cells sit at the base of growth plates at the two ends of bones and fire off cartilage and osteoblasts that lead to the bone lengthening. These cells go away over time, which is why we spring taller as kids and stop growing as adults.
- Irregularity of Healing — One of the mysteries of cell biology involves healing and recovery. Some organs, fueled by their cell networks, heal injuries fairly easily. For instance, if you cut the liver in half, it will grow back. If you fracture a bone, osteoblast cells in the bone will repair it. But other organs don’t heal at all; any damage is permanent. For instance, neurons in the brain and spinal cord will not regenerate and grow back. When kidney cells die, they don’t come back. It’s almost like every organ and cell system has its own Band-Aid for repair and regeneration — they don’t all follow the same pattern when it comes to this.
- Quote (P. 346): “One of the most unusual conundrums of cell biology is that while the early genesis of organs seems to follow a relatively ordered pattern, the maintenance and repair of tissues in adulthood seems idiosyncratic and peculiar to the tissue itself. If you cut the liver in half, the remnant liver cells will divide and grow the liver back to nearly its full size — even in adults. If you fracture a bone, osteoblasts will deposit new bone and repair the fracture — although the process slows down dramatically in older adults. But there are other organs where damage, once done, is permanent. Neurons in the brain and the spinal cord, once they’ve stopped dividing, don’t divide to regenerate neurons. When certain kidney cells die, they don’t come back.”
- Quote (P. 347): “It’s as if every organ, and every cell system, has chosen its own kind of Band-Aid for repair and regeneration. . . To understand injury and repair, then, we have to do it organ by organ and cell by cell.”
- Death: When Rate of Injury Exceeds Rate of Repair — Aging, injury, and death are really a matter of a cell system’s ability to recover vs. the damage being dealt. In younger and healthier people, with younger and healthier cells, the rate of recovery is stronger than the rate of injury or aging. Cells can quickly recover from any damage, unless we’re talking about specific organs where regeneration doesn’t occur (e.g. neurons in the brain). But in older people, with older cells, the rate of injury that comes with aging overwhelms a cell system’s rate of recovery. This eventually leads to death, which is coming for everyone.
- Quote (P. 347): “In cell biological terms, then, it might be easier to imagine injury, or aging, for that matter, more abstractly, as a furious battle between a rate of decay and a rate of repair, with each rate unique for every individual cell, and individual organ. In some organs, injury overwhelms repair. In some organs, repair keeps apace with injury. In yet other organs, there’s a delicate equilibrium between one rate and another.”
- Quote (P. 348): “But death isn’t a flying apart of organs. It is the withering grind of injury set against the ecstasy of healing. . . The central corporals in this pitched battle are cells — cells dying in tissues and organs, and cells regenerating tissues and organs.”
- Quote (P. 348): “Death — the most absolute of absolutes is, in fact, a relative balance between forces of decay and rejuvenation. If you up the balance in one direction — when the rate of injury overwhelms the rate of recovery or regeneration, you fall off the edge.”
- Chapter Takeaway — Aging and death ultimately occur when the different cellular systems throughout the body can no longer repair damage quickly enough. Interestingly, the various cellular systems (organs) in the body have different mechanisms for repair — there isn’t one universal rule that they all follow. For example, when neurons in the brain are damaged or die off, they aren’t repaired or replaced. But a fractured bone will be healed.
Ch. 21: The Selfish Cell
- Cancer: Dysfunctional Cell Division — Cancer is a cell capable of infinite rebirth. No cell has been studied as much, yet we still struggle to beat it. Why? At a basic level, cancer cells don’t follow the rules of regulated cell division. Regulated cell division takes place in our body millions of times every day — it explains why we don’t grow to be giants and why we don’t grow new limbs when we get a cut. In short, our cells know when to stop dividing. But cancer cells don’t. The genes that control cell division and growth mutate, meaning the accelerator and the brakes are permanently broken. This leads to a pile-up of cancer cells, which manifest as tumors. It also causes them to “switch lanes” and go to areas of the body that they aren’t supposed to be. This is spreading (metastasis).
- Quote (P. 350): “But cancer is, in a sense, a disorder of internal homeostasis: its hallmark is that cell division is dysregulated. The genes that control these accelerators and brakes are broken — i.e., mutated — such that proteins that they encode, the regulators of cell division, no longer function in their appropriate contexts. The accelerators are permanently jammed, or the brakes fail permanently. More typically, it is a combination of both events — jammed accelerator genes and snapped brakes — that drives the dysfunctional growth of a cancer cell. The cars speed through the traffic jam, piling up on each other and causing tumors. Or they frantically move into alternate routes, causing metastasis.”
- Cancer: Unique Properties — Another tricky thing about cancer is that each type of cancer has its own unique gene mutations. It is these gene mutations that cause cancer cells to happen. Every cell has genes, but cancer cells have particular gene mutations that cause problems. In other words, breast cancer in one person is not the same, or even all that similar, to another person with breast cancer. They may be characterized the same, but both cases of breast cancer are different and act different, depending on the gene mutations. This trend even extends down to the individual cell level — the individual cells within a cancer tumor can be completely different depending on the person. Even worse, cancer cells can shift to a new set of genes at any time to escape a drug or immunotherapy. When the drug comes at them, they simply scramble to a new set of mutated genes to prevent the drug from treating the screwed up genes it was intended to target.
- Quote (P. 351): “One astonishing feature of cancer is that any individual specimen of cancer has a permutation of mutations that is unique to it. One woman’s breast cancer can have mutations in, say, thirty-two genes; the second woman’s breast cancer can have sixty-three, with only twelve overlapping between the two. The histological, or cellular, appearance of two ‘breast cancers’ may look identical under the pathologist’s microscope. But the two cancers may be genetically different — they behave differently and may require radically different therapies.”
- Quote (P. 351): “Indeed, this heterogeneity of ‘mutational fingerprints’ — the set of mutations carried by an individual cancer cell — reaches down to the individual cell level. The woman’s breast tumor which contained thirty-two mutations in its bulk? It may have an individual cancer cell that has twelve of the thirty-two mutations, and, sitting just adjacent to it, a cell with sixteen of the thirty-two — with some overlapping and some not. Even a single breast tumor, then, is actually a collage of mutant cells — an assemblage of nonidentical diseases.”
- Cancer: Similarity to Stem Cells — Some forms of cancer are marked by cells that behave similarly to stem cells. To recap, stem cells are the ones found in most, if not all, organs that are able to keep pumping out cells. They are the OGs. They replenish the body with new cells when old ones die. Some forms of cancer are fueled by “cancer stem cells” that continuously pump out cancer cells. Some forms of leukemia are like this. Other forms of cancer don’t have cells that act like stem cells. The difference between normal stem cells and cancer stem cells is that cancer stem cells are “locked” into place; they can’t stop pumping deadly cancer cells into the body. Normal stem cells know when to stop pumping out new cells. When it comes to forms of cancer that do have cancer stem cells, the only way to kill the cancer is to cut the head off the snake. If you don’t kill the cancer stem cells, the cancer will keep coming back.
- Quote (P. 355): “These cancer stem cells are like the roots of an invasive plant. You cannot remove the plant without removing the roots — and by the same logic, you cannot kill the cancer without killing the cancer stem cells. . . But the theory that all cancers have stem cells has its challengers.”
- Quote (P. 355): “This much, though, is certain: some cancer cells and stem cells ‘reprogram’ the cell in profound ways. Genes are turned on and off inside the cells to enable their continuous rebirth. The difference is that in cancer, the program is perpetually locked — because the fixity of its mutations doesn’t allow the cell to change its program of continuous division. In normal, healthy stem cells, the program is malleable, because the cell can differentiate — into osteoblasts, or cartilage cells, or red blood cells and neutrophils. Stem cells can change the programs of identity; as I said before, they balance selfishness (self-renewal) with self-sacrifice (differentiation). The cancer cell, in contrast, is trapped — imprisoned in a program of perpetual rebirth. It is the ultimate selfish cell.”
- Cancer: The Warburg Effect — In the 1920s, the German physiologist Otto Warburg discovered that many cancer cells use a fast and cheap method of glucose consumption to generate energy. Cancer cells prefer oxygen-free fermentation rather than the deep-slow burn exhibited in mitochondria, even when there is plenty of oxygen available. Normal cells, in contrast, almost always use a combination of slow and fast burn — oxygen-dependent and independent-mechanisms to generate energy. Cancer cells essentially eat way more glucose than a normal cell. This observation has led to ideas about potentially using this trend against cancer cells.
- Cancer: Using Insulin — Mukhurjee’s lab has found that some forms of cancer like to use insulin — prompted by glucose in the blood — to resist anti-cancer drugs. They essentially use insulin as a defense mechanism against these drugs. What does this mean? Scientists are wondering if they can use cancer’s dependence on certain nutrients — like insulin — against them. In this case, the anticancer drugs are more effective when there is less insulin available, which can be controlled by the patient. Less glucose in the bloodstream, less insulin available for cancer cells to use as defense.
- Chapter Takeaway — Cancer is a horrific disease. Cancer cells are almost immortal; they divide like crazy and are extremely good at changing form to avoid certain drugs and immunotherapies. They also spread to areas of the body that they aren’t supposed to be in. They eat tons of glucose for energy and seem to rely on certain nutrients — like insulin — to help them stay alive.
Ch. 22: The Songs of the Cell
- Cells Work Together — Although we are literally built out of trillions of individual cells, it’s important to keep in mind that these systems of cells throughout the body work together to make the mind and body function. When societies of cells in the body become dysfunctional, it manifests in the body. All health issues and diseases are ultimately the result of cells not working correctly.
- Quote (P. 367): “Systems of cells with specialized functions, communicating with each other through short and long-range messages, can achieve powerful physiological functions that individual cells cannot achieve — for example, the healing of wounds, the signaling of metabolic states, sentience, cognition, homeostasis, immunity. The human body functions as a citizenship of cooperating cells. The disintegration of this citizenship tips us from wellness into disease.”
Epilogue
- Cellular Engineering: Creating New Humans — The future of medicine will involve ‘new humans,’ reengineered with altered cells and genes. This is already happening. Scientists are reengineering cells to create a new pancreas that can be installed in diabetic patients to improve their insulin production. The Mayo Clinic is working on a bio-artificial liver. Scientists have cured patients of sickle cell anemia by reengineering red blood cells and the hemoglobin they carry. Some forms of leukemia are being attacked with beefed up T cells. This is the future of medicine. It’s all about editing our cells to augment and enhance our lives.
- Quote (P. 372): “As I described earlier, a group of researchers including Jeff Karp and Doug Melton are making an ‘artificial pancreas,’ hoping to implant this neo-organ into type 1 diabetics. Two companies, Vertex and ViaCyte, are already enrolling patients to infuse them with pancreatic insulin-producing cells created by making stem cells turn into pancreatic cells. At the Mayo Clinic, scientists are making a bioartificial liver, building them out of liver cells.”
- Sickle Cell Disease — Sickle cell disease is an inherited disorder in which red blood cells shrivel into a sickle shape because of a genetic issue with the hemoglobin molecules they carry around the body. The red blood cells die early due to the issues with hemoglobin, leaving a shortage of healthy red blood cells (sickle cell anemia), and can block blood flow causing extreme pain (sickle cell crisis). Scientists have made a lot of progress toward curing this disease via cellular engineering in which the hemoglobin within the red blood cells is altered.