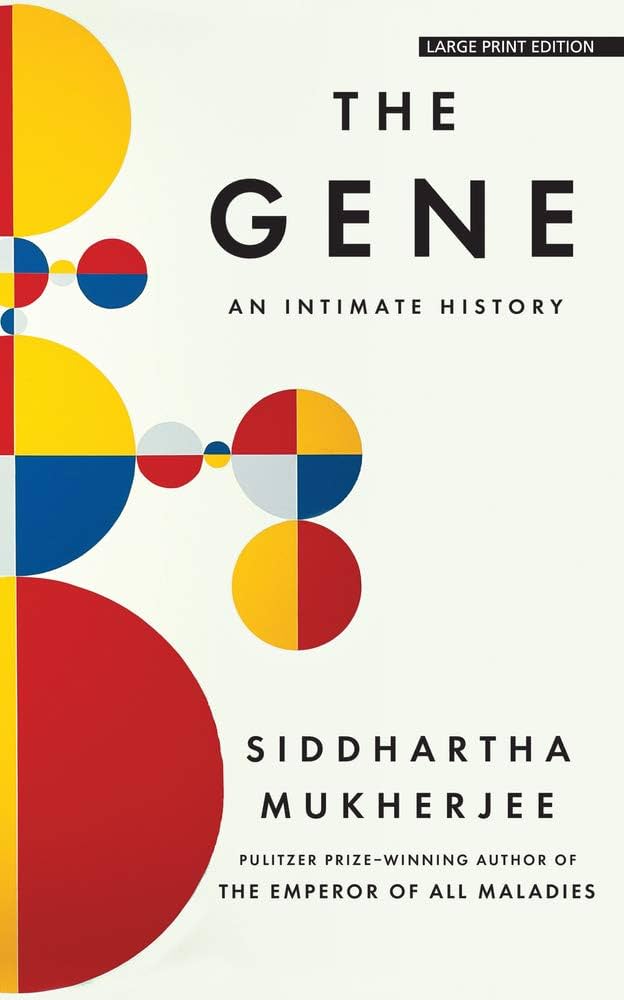
The Gene
Siddhartha Mukherjee
GENRE: Health & Wellness
PAGES: 505
COMPLETED: May 16, 2024
RATING: 




Short Summary
Siddhartha Mukherjee weaves together biology, social history, and the story of his own family to explain the science of genetics and heredity. The Gene takes readers through the history of genetics and describes the technology driving the exciting process of gene editing.
Key Takeaways
Genes: Bringing Organisms to Life — Genes are located in DNA. DNA is is located in the nucleus of every cell in the body. DNA creates RNA molecules, which are essentially copies of DNA that go out and build a chain of amino acids. These amino acids eventually break off and form a protein. Proteins are the workhorses of the body and carry out most of its functions. Essentially — genes, via DNA and RNA, encode proteins, which carry out most of the functions of the body. Certain sequences of DNA coding unlock certain amino acids. These amino acids build the proteins, which then build the organism.
Recombinant DNA & Proteins — The development of recombinant DNA technology in the 1970s and 1980s gave scientists the tools to create and clone genetic hybrids. Through this technology, we unlocked the ability to manufacture proteins in the lab, target millions of biochemical reactions in the body, and develop medications to treat illnesses. Proteins are the hubs of the biological world. All drugs work by binding to certain receptors on proteins and toggling them on and off to achieve certain effects. Among the first medications to be synthesized using recombinant DNA was insulin, which was still being produced from mashed-up cow and pig pancreas at the time.
Exciting Future — The technology to read and change a genome, both before birth and after birth, is here. We already have it. There are still a few major challenges we’re working through, but it won’t be long before gene editing is used to alleviate certain illnesses and prevent diseases entirely (by altering genes in the embryo stage). For a few patients, we’ve already experimented with some of this. Mukherjee refers to these altered humans as “previvors” and “post human.” There will be many of them in the future.
Favorite Quote
“From bacteria to elephants — from red-eyed flies to blue-blooded princes — biological information flowed through living systems in a systematic, archetypal manner: DNA provided instructions to build RNA. RNA provided instructions to build proteins. Proteins ultimately enabled structure and function-bringing genes to life.”
Book Notes 
Prologue
- About the Author — Siddhartha Mukherjee is the author of this book. He is an associate professor of medicine at Columbia University and a cancer physician and researcher. A Rhodes scholar, he graduated from Stanford University, University of Oxford, and Harvard Medical School. He is the author of The Emperor of All Maladies: A Biography of Cancer, winner of the 2011 Pulitzer Prize in general nonfiction; and The Song of the Cell.
- About the Book — This book is about the history of the gene, which is the fundamental unit of heredity and the basic unit of all biological information. It’s about the birth, growth, and future of genetics and the crucial role they play in making each of us who we are.
- Genes: Units of Heredity — Genes reside on chromosomes, which are long structures buried in the nucleus of our cells that contain thousands of genes linked together in chains. We have 46 chromosomes in total — 23 from Mom and 23 from Dad. The entire set of genetic instructions carried by an organism is called its genome. The genome is basically a giant encyclopedia that outlines all genes, with footnotes, annotations, instructions, and references. The human genome contains between 21-23,000 genes that provide the master instructions to build, repair, and maintain humans. To understand an organism, you have to first understand its genes.
- Altering Genes — As we continue to learn more and more about genes, our ability to manipulate them is advancing. Gene editing is already being used in certain ways, and it will only continue to grow in usage and prominence going forward. Gene editing will likely be a major part of the future of medicine. But with it comes many questions about what is ethical and what isn’t. Gene editing has she potential to do a lot of good, but it’s also a bit scary.
- Quote (P. 12): “I mean the following: in just the last four years — between 2012 and 2016 — we have invented technologies that allow us to change human genomes intentionally and permanently. . . We can now ‘read’ human genomes, and we can ‘write’ human genomes in a manner inconceivable just three or four years ago.”
Ch. 1: The Walled Garden
- Confusion About Likeness — In the BC days, there was major confusion about why children looked like their parents. Pythagoras and Plato had various theories. Pythagoras thought babies were tiny things inside of a man’s sperm that grew when transitioned into a women’s body. This was the idea of preformation, which is obviously wrong. Aristotle later dismissed the idea of preformation, instead championing the idea that heredity was about the transmission of information. In his view, information was then used to build an organism from scratch. This is much closer to the truth than preformation.
- Chapter Takeaway — There was major confusion about genetics and heredity in the early days. Nobody really knew how it worked.
Ch. 2: The Mystery of Mysteries
- Charles Darwin: Moving Away From Divinity — In the 1830s, Charles Darwin traveled from England to the Galápagos Islands, where he collected all kinds of fossils on his trip and observed different species of finches. After examining the fossils and thinking about the differences in beaks among the finches, Darwin began to think that nature was a series of cause and effects. He began to think that plants and animals descended from earlier forms through a process of gradual, continuous change. This was radically different than the dominant thinking of the time, which was that God created the world and everything happened at once. He began to think of nature as process and as progression.
- Quote (P. 35): “Like languages, like landscapes, like the slowly cooling cosmos, perhaps the animals and plants had descended from earlier forms through a process of gradual, continuous change. It was, Darwin knew, an explicitly profane diagram. The Christian concept of speciation placed God firmly at the epicenter; all animals created by Him sprayed outward from the moment of creation. In Darwin’s drawing, there was no center.”
- Darwin: Evolution & Natural Selection — In 1859, two decades after visiting the Galápagos Islands, Darwin published his book On the Origin of Species by Means of Natural Selection. In it, Darwin argued that species progress and evolve over time, with the traits, skills, and features that are best suited for the environment winning out. Animals within a species that have traits, skills, features, etc. that are not best suited for the environment they’re living in die without reproducing. As a result, the strongest survive and reproduce, creating more of those suitable traits, skills, features, etc. This was one of Darwin’s central claims in his theory of natural selection. Survival of the fittest. The implication of Darwin’s book was that we humans basically evolved from apes, a very controversial stance at the time.
- Quote (P. 37): “Darwin now had the skeletal sketch of his master theory. When animals reproduce, they produce variants that differ from the parents. Individuals within a species are constantly competing for scarce resources. When these resources form a critical bottleneck — during a famine, for instance — a variant better adapted for an environment is ‘naturally selected.’ The best adapted — the ‘fittest’ — survive (the phrase ‘survival of the fittest’ was borrowed from the Malthusian economist Herbert Spencer). These survivors then reproduce to make more of their kind, thereby driving evolutionary change within a species.”
- Chapter Takeaway — In the 1830s-50s, Charles Darwin began to piece together his theory of natural selection and evolution after digging for fossils on a trip to the Galápagos Islands.
Ch. 3: The "Very Wide Bank"
- Heredity: Instructions & Adaptation — Hereditary traits were thought to be passed down from parents to offspring as instructions, just like a message or story might be passed. As a certain species adapts to its environment, it develops slight variants in order to survive (e.g. a finch’s beak). These variations are then passed down to offspring via hereditary “instructions.”
- Quote (P. 42): “The immediate appeal of [Jean Baptiste] Lamarck’s idea was that it offered a reassuring story of progress: all animals were progressively adapting to their environments, and thus progressively slouching along an evolutionary ladder toward perfection. Evolution and adaptation were bundled together into one continuous mechanism: adaptation was evolution.”
- Darwin & “Pangenesis” — In the 1860s, Darwin began writing another book, this one about how genes are passed down. His first book was more about the mere presence of genetics. He called his theory “pangenesis,” which was basically his belief that all cells in the body carried “code” (what we now know is DNA), or instructions, for building the part of the body that they resided in. For example, hand cells contain code that instructs a baby’s hand how to grow. When reproduction occurs, the instructions from the male and female body meet in the embryo and blend together like paints or colors. This idea was later debunked; in reality, there is no blending. An offspring inherits genes from each parent individually.
- Chapter Takeaway — Darwin’s idea of pangenesis significantly advanced our understanding of heredity.
Ch. 4: The "Flowers He Loved"
- Playing With Peas — In the 1850s, an Austrian man named Gregor Mendel, after twice failing a teaching credential exam, began playing around with peas in his garden. He was interested in uncovering the mysteries of heredity. In is garden, he mixed and matched different peas (e.g. tall with short, yellow with green, etc.) to see what kind of outcomes he could get. He did this for eight years and found that he could produce hybrid peas that resembled one of the parent peas.
- Dominant & Recessive Traits — In analyzing his hybrid peas, Mendel found that each of them had dominant and recessive traits. In other words, a hybrid pea would resemble one of its parents depending on the dominant or recessive traits it inherited. This discovery that offspring peas have dominant and recessive traits directly went against the dominant thinking at the time, which was that genes blended together. Both sets of traits would be present, but only one set would assert itself (the dominate). There were no intermediate features as the blending theory suggested.
- Quote (P. 52): “Heredity, Mendel’s experiments implied, could only be explained by the passage of discrete pieces of information from parents to offspring. Sperm brought one copy of this information (an allele); the egg brought the other copy (a second allele); an organism thus inherited one allele from each parent. When that organism generated sperm or eggs, the alleles were split up again — one was passed to the sperm, and one to the egg, only to become combined in the next generation. One allele might ‘dominate’ the other when both were present. When the dominant allele was present, the recessive allele seemed to disappear, but when a plant received two recessive alleles, the allele reiterated its character. Throughout, the information carried by an individual allele remained indivisible.”
- Long Wait — These pea experiments essentially led Mendel to the discovery of genes, even though he never officially called these units of information “genes” during his lifetime. After Mendel published his pea findings in a monumental paper in 1865, nobody really acted on the information. It was bizarre. The paper was cited just four times between 1866-1900, virtually disappearing from scientific literature. Such a huge breakthrough was followed by almost complete silence. It was odd.
Ch. 5: "A Certain Mendel"
- Darwin & Mendel — Although Darwin and Mendel never formally worked together, their findings were linked. Darwin observed the fact that species evolved over time. But the mechanical ways that these small, evolutionary changes took place over time were unknown to Darwin. Mendel’s discovery of genes and how they serve as an organism’s assembly instructions (heredity) provided the answer Darwin needed. How did genes work? Messages from cells throughout the body assemble in sperm as a manual for building an organism then combine with the genes in the woman’s eggs. The offspring then inherits genes from each of the parents, with one being more dominant than the other in each area of the body.
- Mutations — Again, Darwin was responsible for observing that species evolve over time, but he didn’t know exactly how. Mendel’s discoveries on genes filled that knowledge gap. Hugo de Vries also contributed to filling the knowledge gap by pointing out that genetic mutations are responsible subtle changes to a species over time. Mutations create variants in nature: short-beaked finches, long-necked antelopes, etc. As each species tries to survive in current conditions, they develop these slight mutations, which get passed down to later generations. This process drives evolution.
Ch. 6: Eugenics
- Eugenics — As our understanding of genes and heredity advanced in the mid-late 1800s and early 1900s, the idea of trying to control which traits survived and multiplied became a real topic. This idea of selective breeding was given the name ‘eugenics’. It was basically the attempt to improve the human race via artificial selection of genetic traits and purposeful breeding of humans who carry those traits.
- Genes: Units of Heredity — As Mendel found, genes are essentially units of heredity. Each of these units contains code for how to build a human. Half of an organism’s genes come from the dad, and half come from the mom. Each parent passes down genes, or instructions, which are then decoded to make a human. Interestingly, Mendel didn’t come up with the word ‘gene’; the name was later assigned in 1909 by William Johannsen. At that time, however, it was still very unclear what a gene was, including how it worked, what it was made of, etc. All scientists knew at the time was what Mendel had discovered: genes are responsible for the changes in a species over time.
- Quote (P. 69): “A child was an ancestral composite, but a supremely simple one: one-half from the mother, one-half from the father. Each parent contributed a set of instructions, which were decoded to create a child.”
- Chapter Takeaway — Eugenics was introduced in 19th century and is the idea of trying to identify and multiply the best traits in a species via intentional breeding.
Ch. 7: "Three Generations of Imbeciles Is Enough"
- Buck v. Bell — As the debates around eugenics grew, people who were deemed to have inferior genetics in some way were sent to a colony in Lynchburg, Virginia to basically be detained. Among the people sent to this colony was Carrie Buck, who in 1927 was involuntarily sterilized after the Supreme Court ruled against her in Buck v. Bell. All of this took place roughly 60 years after Mendel discovered genes. Buck v. Bell only ignited the ongoing discussion about genetics and eugenics. In 1927, the state of Indiana passed a law to sterilize “confirmed criminals, idiots, imbeciles, and rapists.” Other states followed with similar legal measures to sterilize men and women who were deemed to be genetically inferior.
Ch. 8: "Abhed"
- Genes Carried On Chromosomes — Genes are physically located on chromosomes. In 1905, biologist Nettie Stevens demonstrated that “maleness” in mealworms was determined by the Y chromosome since the Y chromosome was only ever present in male embryos, but never female ones. Females have the X chromosome, which is a little longer and less stubby than the Y chromosome under a microscope. Having pinpointed the location of gender-carrying genes in a single chromosome, Stevens proposed that all genes might be carried on chromosomes.
- Genes Linked On Chromosomes — Imagine a chromosome as a string. Now imagine genes as pearls strung along on that string. That’s the relationship genes and chromosomes have. In the early 1900s, Thomas Morgan, studying fruit flies in his lab, discovered that genes located close together on the same chromosome were highly correlated. For example, in humans the genes for blond hair and blue eyes, and dark hair and brown eyes, are packed together closely on the same chromosome and therefore are very tightly linked. Genes that are not very correlated with each other are like two pearls that are very far apart on the string (chromosome). In this way, genes don’t move independently; genes are packets of code that are located on chromosomes, which are packed into our cells.
- Quote (P. 95): “Morgan had discovered an important modification to Mendel’s laws. Genes did not travel separately; instead, they moved in packs. Packets of information were themselves packaged — into chromosomes, and ultimately in cells.”
- Quote (P. 96): “Morgan’s experiments had established that genes that were physically linked to each other on the same chromosome were inherited together. If the gene that produces blue eyes (call it B) is linked to a gene that produces blond hair (Bl), then children with blond hair will inevitably tend to inherit blue eyes (the example is hypothetical, but the principle that it illustrates is true).”
- Chapter Takeaway — Genes are physically located on chromosomes, which are packed into our cells. The closer two genes are to each other on the chromosome, the more correlated they are.
Ch. 9: Truths and Reconciliations
- More About Genes — To reiterate, genes carry the information and the code (the instructions) to build an organism. They are responsible for physical features, like how you look), as well as mental and emotional features, like how you think and feel. It’s important to note, however, that genes are not the end all, be all. Certain environmental conditions (e.g. upbringing) and triggers also play a role. But genes are the central currency for biological information.
- Genotypes & Phenotypes — There are two ways to classify genes: genotypes and phenotypes. A genome is an organism’s genetic composition. It can refer to one gene, a configuration of genes, or even an entire genome. A phenotype refers to an organism’s physical or biological attributes and characteristics — eye color, height, facial features, etc.
- Genotypes & Phenotypes: Multiple Factors — Just because a certain gene is part of an organism’s genotype does not mean that it automatically results in a corresponding phenotype. In reality, there are many factors that contribute to an organism’s physical features, including environmental cues and triggers, as well as pure luck. As an example, a mutated BRCA1 gene increases the risk for breast cancer in humans — but not all women who have this gene end up developing the cancer. The morale of the story is this: you can’t just predict how an organism will turn out purely based on its genotype. Although genes certainly are a great indicator, there are several other factors that are also in play. This formula sums it up:
- Genotype + Environment + Triggers + Chance = Phenotype
- Multiple Genes Affect Phenotypes — Genes do not lead to certain features (e.g. height, intelligences beauty, etc.) in a one-to-one manner. Rather multiple genes are usually in play with phenotypes, or physical features in an organism. This is why proponents of eugenics were so misinformed; they believed they could manipulate outcomes in a race for centuries to come just by eliminating certain phenotypes that they determined to be inferior (e.g. skin color). In reality, there are multiple genes, and multiple configurations of those genes along the chromosome, that lead to the various features each of us present. There are also many other factors — like environment, other triggers, and luck — that have an impact.
- Quote (P. 110): “Eugenicists such as Galton had hoped to select complex phenotypes — intelligence, height, beauty, and moral rectitude — as a biological shortcut to enrich genes for intelligence, height, beauty, and morality. But a phenotype was not determined by one gene in a one-to-one manner.”
- Chapter Takeaway — A genotype reveals the collection of genes an organism possesses. A phenotype is a particular feature of an organism. Genotypes do not automatically lead to certain phenotypes; the environment that surrounds the organism, other triggers, and pure luck and chance also play a role in addition to genotypes.
Ch. 10: Transformation
- Transferring Genes — In the 1920s, scientists discovered that genes can be transferred between organisms in some cases. For example, bacteria can transfer genes, and there were several studies that proved this. It’s not possible in humans, but it would be like two friends swapping genes to get different eye colors. This finding was important because it was the first time scientists were able to confirm that genes are a chemical substance. Up to this point, scientists knew that genes existed and understood what they do from a hereditary standpoint, but they still had no clue what genes looked like, how they were constructed, etc. This discovery allowed scientists to intercept genes during the transfer between bacteria in order to study their structure and chemical makeup.
- Manipulation of Genes — Around this same time, the 1920s, other researchers like Hermann Muller performed experiments proving that genes can be altered fairly easily. His experiments used radiology from x-rays to change the genetic makeup of flies. The experiments launched him into international fame because they proved that genes are not fixed, permanent things. They are pliable and can be manipulated, which created a lot of excitement among proponents of eugenics.
- Chapter Takeaway — A series of experiments in the 1920s and 1930s uncovered two critical pieces of information about genes: (i) genes can be transferred between organisms in some cases (bacteria can transmit genes to each other); and (ii) genes can be manipulated by outside forces.
Ch. 11: Lebensunwertes Leben
- Adolf Hitler Rises to Power — Eugenics continued to take the world by storm as new findings about genes were uncovered. In Germany, things were getting really creepy. People there were becoming obsessed with eliminating genes, and races of people, that they deemed inferior. At the forefront of that obsession was Adolf Hitler, who in January 1933 was appointed chancellor of Germany. In March of that year, the German parliament endorsed the Enabling Act, which gave Hitler unprecedented power to enact laws without parliamentary involvement. This set the stage for the horror that was to come with the Nazis.
- “Gene Cleansing” — The Nazis, led by Hitler, were obsessed with “cleansing” Germany of inferior genetics. They started small in 1933 by forcibly sterilizing criminals and people they deemed to be genetically “defective” in some way. But things quickly turned sinister. By 1939, Hitler and the Nazis began outright murdering people. The killing began with “defective” children under the age of three after Richard and Lina Kretschmar asked Hitler for permission to euthanize their child, Gerhard. Hitler approved, and everything avalanched from there. Soon “defective” adolescents and adults were being murdered.
- Extermination Camps — To carry out the murders, the Nazis set up extermination camps with airtight chambers and gassed people to death with carbon monoxide. Throughout this crusade, the Nazis targeted Jews. They wanted to eliminate the Jewish population. In total, Hitler’s genetic cleansing program exterminated 6 million Jews during the Holocaust.
- Josef Mengale: The Angel of Death — In 1936, the University of Munich, an institution supported by Hitler, awarded a PhD to a young medical researcher for his thesis concerning the “racial morphology” of the human jaw — an attempt to prove that the anatomy of the jaw was racially determined and genetically inherited. The newly minted “human geneticist,” Josef Mengele, would soon rise to become the most epically perverse of Nazi researchers, whose experiments on prisoners would earn him the title Angel of Death.
- Chapter Takeaway — Obsession with eugenics and “gene cleansing” quickly escalated into “ethnic cleansing,” driving Hitler and the Nazis to kill more than 6 million Jewish people during the Holocaust.
Ch. 12: "That Stupid Molecule"
- Chromatin — By the 1940s, scientists had started opening cells and studying their internal parts. One component of cells that they discovered was chromatin, a structure where genes resided that was made of two types of chemicals: proteins and nucleic acids. The primary function of chromatin is to package long DNA molecules into more compact, denser structures. More on these two chemicals inside of chromatin:
- Proteins — Proteins are made of chains of amino acids and carry out the bulk of functions in the cell. They are the workhorses of the cell. Cells depend on chemical reactions to live: during respiration, for example, sugar combines chemically with oxygen to make carbon dioxide and energy. None of these reactions occur on their own; proteins are what control and regulate them so we can live. Proteins also form the structural components of the cell: filaments of hair, nails, cartilage — these are all aided by proteins. Proteins are also twisted into other shapes to form receptors, hormones, and signaling molecules, allowing cells to communicate with each other.
- Quote (P. 134): “Nearly every cellular function — metabolism, respiration, cell division, self-defense, waste disposal, secretion, signaling, growth, even cellular death — requires proteins. They are the workhorses of the biochemical world.”
- Quote (P. 141): “Proteins enabled chemical reactions, speeding and controlling the pace of biochemical processes, thereby acting as the switchboards of the biological world.”
- Nucleic Acids — Nucleic acid is a chemical that comes in two forms: DNA and RNA. Both are long chains made of four components, called bases, strung together along a string-like chain. In DNA, the four bases strung along on the chain are adenine, guanine, cytosine, and thymine — abbreviated A, T G, C. In RNA, the thymine is switched into uracil — abbreviated A, C, G, U. The string-like chain in DNA and RNA is made of a chain of sugars. In DNA, the sugar is a chemical called deoxyribose — hence Deoxyribo-Nucleic Acid (DNA). In RNA, the sugar is ribose — hence Ribo-Nucleic Acid (RNA).
- Proteins — Proteins are made of chains of amino acids and carry out the bulk of functions in the cell. They are the workhorses of the cell. Cells depend on chemical reactions to live: during respiration, for example, sugar combines chemically with oxygen to make carbon dioxide and energy. None of these reactions occur on their own; proteins are what control and regulate them so we can live. Proteins also form the structural components of the cell: filaments of hair, nails, cartilage — these are all aided by proteins. Proteins are also twisted into other shapes to form receptors, hormones, and signaling molecules, allowing cells to communicate with each other.
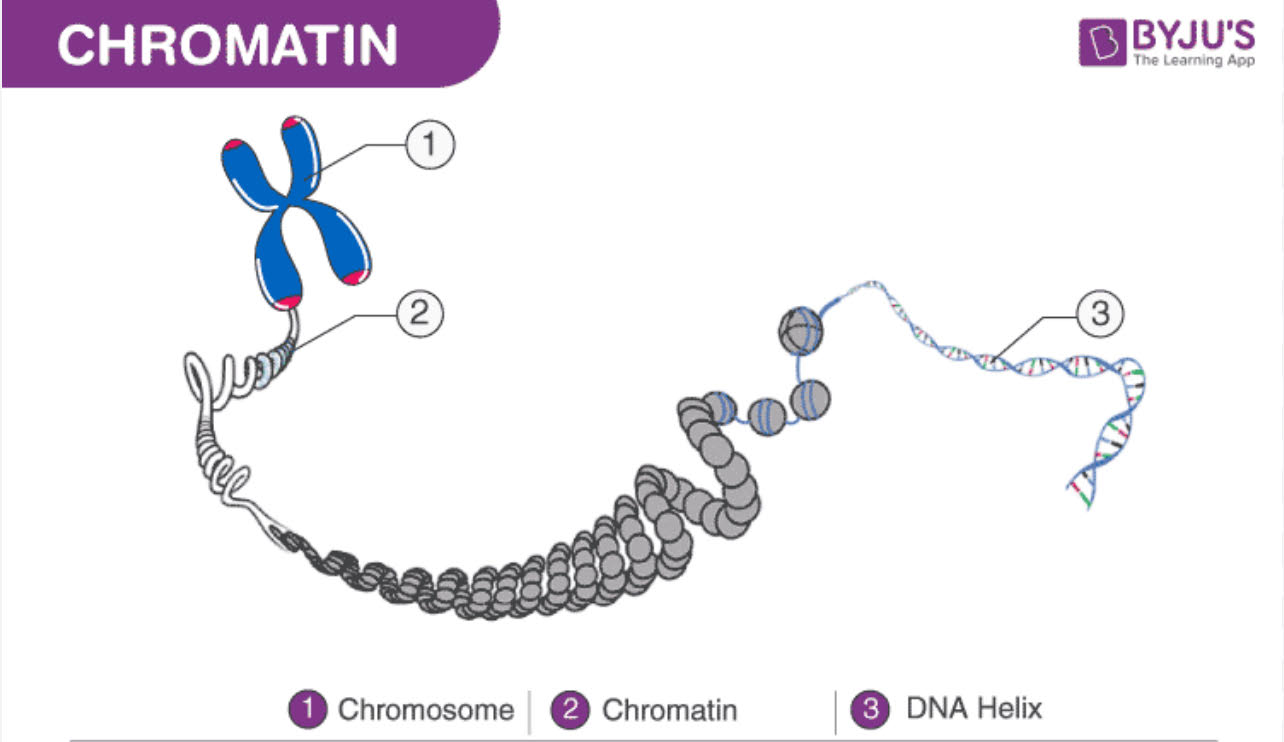
- DNA: The Carrier of Genes — In the 1940s, Oswald Avery conducted a series of thorough experiments and research that proved DNA is the molecule that carries genetic information. This had been a mystery for decades. Scientists knew what genes did, but they did not know anything about the material substance of genes. Avery’s paper on DNA in 1944 was a monumental moment in the history of genetics.
- Chapter Takeaway — DNA is the carrier of genetic information. Oswald Avery made this discovery by intercepting the transfer of genes between bacteria and studying the internal components of chromatin.
Ch. 13: "Important Biological Objects Come in Pairs"
- Race to Uncover DNA’s Structure — In the 1940s and 1950s, several prominent scientists were engaged in a fierce race to uncover the structure of DNA. By this point, Oswald Avery and other scientists had shown that DNA is the carrier of genes and genetic information. But the actual structure and molecular composition of DNA was still unknown. There was a huge race to figure out the structure of DNA, with several scientists simultaneously conducting research and assembling models in an effort to be the first to discover it. It took a very long time to figure it out, and it was an incredibly tough task.
- DNA: The Double Helix — James Watson and Francis Crick in 1953 finally solved the mystery of DNA’s structure. DNA, as they showed, is a molecule set in the shape of a helix in which each individual strand is comprised of a backbone and four “bases” that are attached to the backbone and stick out of it like the teeth on a zipper. The backbone is made of sugars and phosphates, while the bases are made of adenine, guanine, cytosine, and thymine — abbreviated A, G, C, T. In order to connect and make a double helix, the “bases” of each individual DNA strand merge together, with adenine (A) connecting with thymine (T) and guanine (G) connecting with cytosine (C). Watson and Crick were awarded the Nobel Prize in 1962 for uncovering the molecular structure of DNA.
- Quote (P. 157): “Each strand of DNA, recall, is a long sequence of ‘bases’ — A, T, G, and C. The bases are linked together by the sugar-phosphate backbone. The backbone twists on the outside, forming a spiral. The bases face in, like treads in a circular staircase. The opposite strand contains the opposing bases: A matched with T and G matched with C. Thus, both strands contain the same information — except in a complementary sense: each is a ‘reflection’, or echo, of the other (the more appropriate analogy is a yin-and-yang structure). Molecular forces between the A:T and G:C pairs lock the two strands together, as in a zipper. A double helix of DNA can thus be envisioned as a code written with four alphabets — ATGCCCTACGGGCCCATCG… — forever entwined with its mirror-image code.”
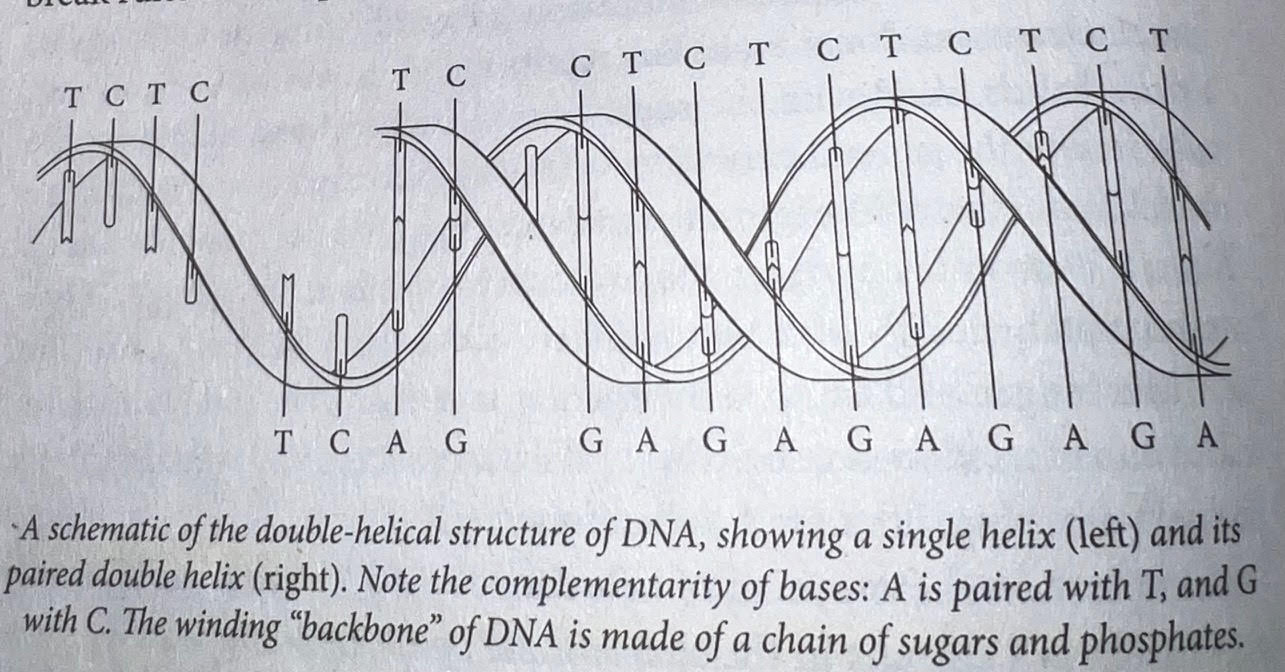
- Components of DNA — DNA is stored chromosomes located in the nucleus of every one of the 37+ trillion cells in our body, and there is about 3-6 feet of DNA contained in each cell (if you decided to string it out). That’s a lot of DNA! Within DNA are units called genes. Genes provide instructions for building proteins (and therefore us). Most of the useful things in the body are proteins. Some speed up chemical changes within cells and are known as enzymes. Others convey chemical changes and are called hormones. Others attack bad stuff in the body and are called antibodies. Interestingly, all humans share 99.9% of their DNA, yet no two humans are alike. There are 3-4 million places in our DNA where everybody’s genes are different, and that’s why we are all different.
- Chapter Takeaway — The discovery of DNA’s molecular structure by James Watson and Francis Crick in 1953 put an end to one of the great mysteries of human biology. For decades, going back to Gregor Mendel, scientists understood that genes were the reason for heredity and the passing down of traits from generation to generation. But they had no idea where genes were stored or what DNA really was. Watson and Crick’s modeling of the double helix of DNA remains one of the great moments in human history, but it also opened up a flood of additional questions and mysteries.
Ch. 14: "That Damned, Elusive Pimpernel"
- How Genes Function: A Start — Even after Watson and Crick discovered the double helix structure of DNA, there was still a lot of mystery regarding how genes actually delivered their code and produced physical features in an organism’s body. In 1945, George Beadle and Edward Tatum got the ball rolling by finding that genes deliver code and instruction to enzymes (proteins that act as master builders) in every cell, which then produce the physical traits that the genes prescribed. This theory helped explain why some fruit flies in the lab, for example, had white eyes rather than red — if a mutation disables even one protein enzyme, then things become disrupted and you get different outcomes (white eyes instead of red). In this way, genes carry instructions for proteins, which then build the organism. Although Beadle and Tatum won a Nobel Prize for this “missing ingredient” theory, genes are more than a set of protein-building instructions. These experiments put scientists on the right track though.
- Quote (P. 163): “The ‘missing ingredient’ experiment propelled Beadle and Tatum toward a new understanding of genes. Every mutant, they noted, was missing a single metabolic function, corresponding to the activity of a single protein enzyme. And genetic crosses revealed that every mutant was defective in only one gene.”
- Quote (P. 163): “‘A gene’, Beadle wrote in 1945, ‘can be visualized as directing the final configuration of a protein molecule.’ This was the ‘action of the gene’ that a generation of biologists had been trying to comprehend: a gene ‘acts’ by encoding information to build a protein, and the protein actualizes the form or function of the organism.”
- About Proteins — A protein is created after amino acids, which are usually made up of 20 simple chemicals (there can be more or less depending on the protein; insulin has more than 50), stack up together and create a chain. Unlike DNA, which is fixed in the position of a double helix, proteins are very versatile and can twist and turn in space very easily. This shape-shifting ability allows proteins to carry out a wide range of diverse functions in cells that make them extremely valuable and useful. Some proteins speed up chemical changes within cells and are known as enzymes. Others convey chemical changes and are called hormones. Others attack bad stuff in the body and are called antibodies.
- Quote (P. 163): “This shape-acquiring ability allows proteins to execute diverse functions in cells. They can exist as long, stretchable fibers in muscle (myosin). They can become globular in shape and enable chemical reactions — i.e., enzymes (DNA polymerase). They can bind colored chemicals and become pigments in the eye, or in a flower. Twisted into saddle clasps, they can act as transporters for other molecules (hemoglobin). They can specify how a nerve cell communicates with another nerve cell and thus become the arbiters of normal cognition and neural development.”
- RNA: Gene Messengers — How does the message from genes (which are located in DNA, and DNA is stored inside the chromosomes inside of the nucleus of cells) make its way over to a different part of the cell (cytoplasm) to build proteins? Scientists began to think there was a “messenger” involved. That messenger was identified as RNA, DNA’s molecular cousin with bases of A, G, C, and U (DNA has a ‘T’ rather than a ‘U’). Essentially, the code from genes in DNA is copied into RNA molecules, which shuttle the instructions and translate them into amino acids, which stack together in a chain to form proteins. Proteins then build the organism based on the gene’s instructions.
- Quote (P. 166): “Notably, Brenner and Jacob later discovered the messenger RNA was a facsimile of the DNA chain — a copy made from the original. The RNA copy of a gene then moved from the nucleus to the cytosol, where its message was decoded to build a protein.”
- Quote (P. 181): “Units of hereditary information, encoded in DNA and packaged on chromosomes, are transmitted through sperm and egg into an embryo, and from the embryo to every living cell in an organism’s body. These units encode messages to build proteins — and the messages and proteins, in turn, enable the form and function of a living organism.”
- RNA: Protein Builders — The bullet above explains how the genetic messages from DNA become proteins, but how is the message from RNA actually translated and used to build proteins? It has a lot to do with the amino acids used to build proteins (the amount of amino acids depends on the protein; insulin has more than 50). Essentially the four bases of DNA are sequenced in some way depending on the gene (e.g. ACT-GAC-CAC-GTG) and copied into RNA, which then translates the DNA’s coding to build a chain of amino acids. These amino acids stack together and eventually form a protein. Certain sequences of DNA coding unlock certain amino acids. These amino acids build the proteins, which then build the organism.
- Quote (P. 168): “A particular sequence of DNA-ACT-GAC-CAC-GTG-was therefore used to build an RNA chain, and the RNA chain was translated into a chain of amino acids, ultimately leading to the construction of a protein.”
- Quote (P. 169): “From bacteria to elephants — from red-eyed flies to blue-blooded princes — biological information flowed through living systems in a systematic, archetypal manner: DNA provided instructions to build RNA. RNA provided instructions to build proteins. Proteins ultimately enabled structure and function-bringing genes to life.”
- Quote (P. 215): “The ‘alphabet’ of genes has only four letters: the four bases of DNA — A, C, G, and T. The ‘vocabulary’ consists of the triplet code: three bases of DNA are read together to encode one amino acid in a protein; ACT encodes Threonine, CAT encodes Histidine, GGT encodes Glycine, and so forth. A protein is the ‘sentence’ encoded by a gene, using alphabets strung together in a chain (ACT-CAT-GGT encodes Threonine-Histidine-Glycine). And the regulation of genes [via turning genes ‘on’ and ‘off’]. . . creates a context for these words and sentences to generate meaning.”
- Quote (P. 325): “The genetic code is simple: DNA is used to build RNA, and RNA is used to be build a protein. A triplet of bases in DNA specifies one amino acid in the protein.”
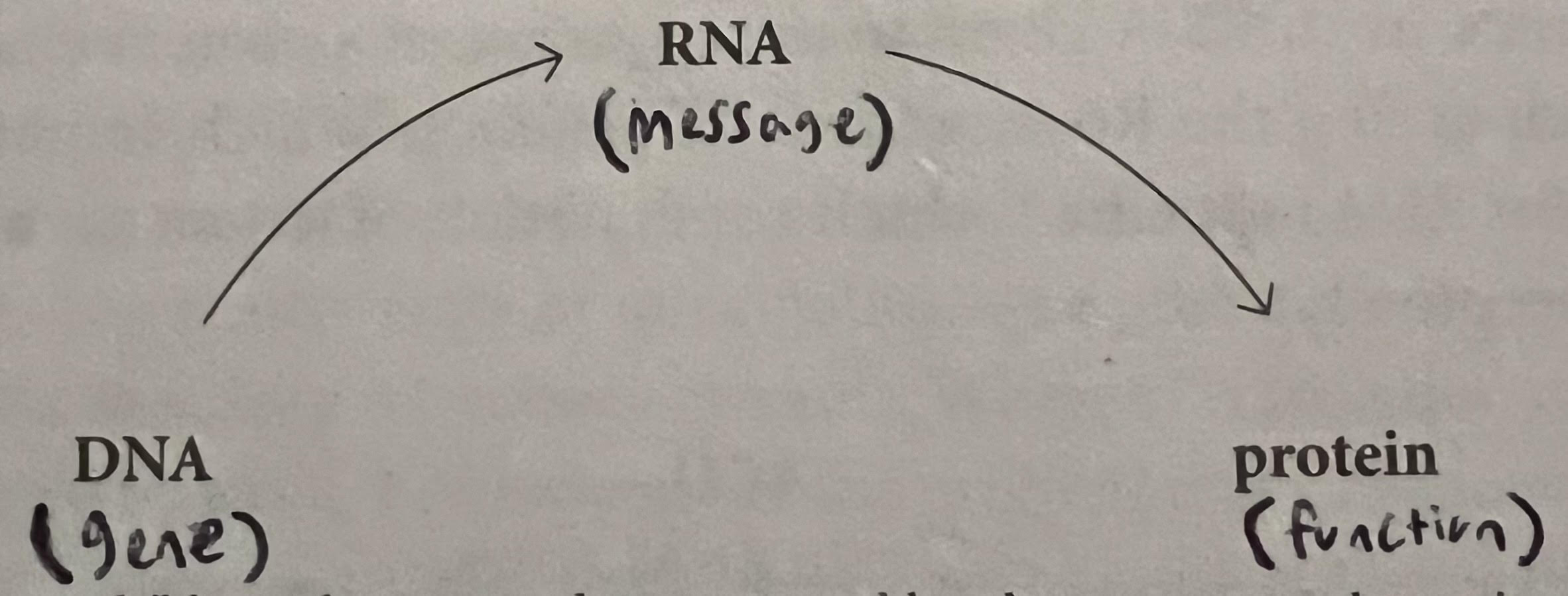
- Case Study: Sickle Cell Anemia — To tie all of this together, look at sickle cell anemia, which is a hereditary condition in which red blood cells (which are usually in the shape of a disk) shrivel up into the shape of a crescent, especially in the absence of oxygen (think Ryan Clark playing in Denver). These crescents, unable to glide smoothly through capillaries and veins, then plug up microscopic clots in the body to create severe pain. Sickle cell anemia is caused by one altered amino acid. This altered amino acid is caused by one tiny incorrect gene sequence in the person’s DNA (GAG is incorrectly sequenced as GTG). Because the DNA sequence is off, the message from RNA creates an incorrect amino acid, which builds an incorrect protein, which causes the condition of sickle cell anemia. When certain proteins are incorrect, these type of conditions (e.g. cancer, disease, miniature limbs, etc.) can happen. It all ties back to genes and genetic coding/sequencing. If that is off, everything else is off.
- Quote (P. 171): “A change in the sequence of a gene caused the change in the sequence of a protein; that warped its shape; that shrank a cell; that clogged a vein; that jammed the flow; that racked the body (that genes built). Gene, protein, function, and fate were strung in a chain: one chemical alteration in one base pair in DNA was sufficient to ‘encode’ a radical change in human fate.”
- Chapter Takeaway — Genes are stored in DNA, which is stored in chromosomes, which are stored in the nucleus of cells. DNA builds proteins, which build the organism. The “messenger” that carries DNA’s code to the cytoplasm for protein building is called RNA.
Ch. 15: Regulation, Replication, Recombination
- DNA: Regulation — DNA is not just a passive blueprint for building an organism. Even though every cell contains the same exact genes, some genes are activated (turned on) and some are repressed (turned off), which explains why certain cells react in certain ways despite the fact that they all contain the exact same genetic coding. As an example, sickle cell anemia is the result of a certain gene irregularity in red blood cells only. This irregularity in gene sequencing exists in every cell of the patient’s body, but it is only activated in red blood cells. What causes some genes to be activated or suppressed in certain cells? The answer is proteins (or enzymes). Proteins are like the controllers at a master switchboard — they determine which genes are activated and which are not. DNA regulation is really important; diseases can arise from the inability to turn the right gene on or off in the appropriate cell at the right time.
- Quote (P. 177): “Proteins act as regulatory sensors, or master switches, in this process — turning on and turning off genes, or even combinations of genes, in a coordinate manner.”
- Quote (P. 183): “That genes could be regulated — i.e., turned ‘on’ and ‘off’ by particular cues — deepened the understanding of how genes function in time and space to specify the unique features of distinct cells.”
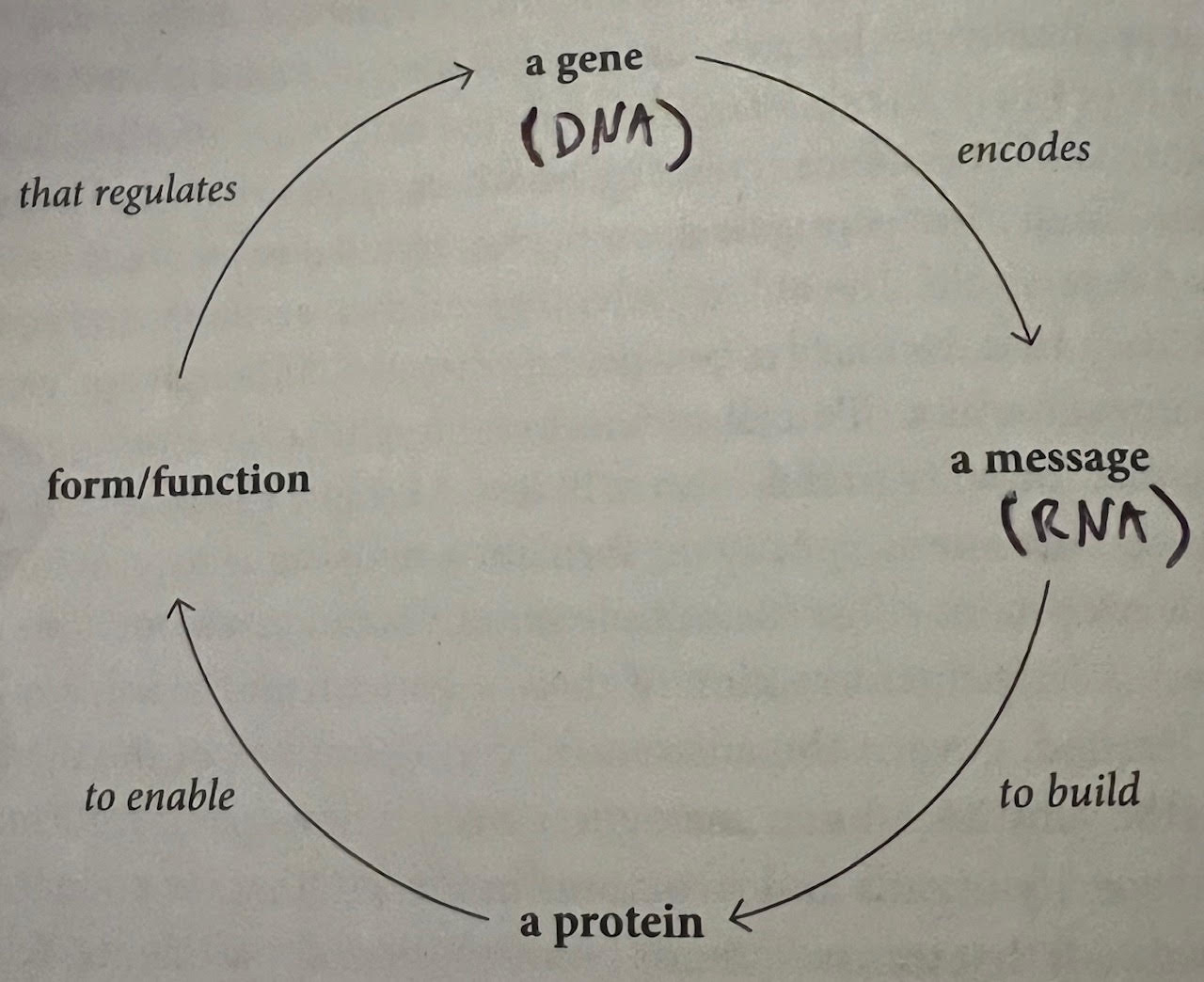
- DNA: Replication — How are genes replicated when a cell divides, or when a sperm or egg is generated? The answer is DNA polymerase, a protein enzyme that enables DNA to replicate. Interestingly, DNA polymerase is itself the product of a gene. Built into every genome are the codes for proteins that allow that genome to replicate. Going back to the bullet above, this can be a problem if DNA polymerase and other proteins that allow DNA to replicate go rogue, causing a furious and unwarranted rate of DNA and cell replication. This is the mark of one of the worst diseases known to man, cancer. Cancer is the result of incorrect gene coding, and it becomes deadly when it starts to replicate uncontrollably and spread throughout the body. DNA replication is really important; it explains how a multicellular organism emerges from a single cell, and errors in replication explain how an illness or disease (e.g. cancer) might arise in a previously unaffected family.
- Quote (P. 180): “There is a recursion here that is worth noting: like all proteins, DNA polymerase, the enzyme that enables DNA to replicate, is itself the product of a gene. Built into every genome, then, are the codes for proteins that will allow that genome to reproduce. This additional layer of complexity — that DNA encodes a protein that allows DNA to replicate — is important because it provides a critical node for regulation. DNA replication can be turned on and turned off by other signals and regulators, such as the age or the nutritional status of a cell, thus allowing cells to make DNA copies only when they are ready to divide. This scheme has a collateral rub: when the regulators themselves go rogue, nothing can stop a cell from replicating continuously. That, as we will soon learn, is the ultimate disease of malfunctioning genes — cancer.”
- DNA: Recombination — Another question around genes is this: for evolution to occur, there has to be variation in genes — so how does that occur? An example: over time, the offspring of finches that Darwin studied had to develop different beaks to survive. So how do these genetic variations take place? The following mechanisms can occur:
- Mutation — One mechanism involves mutation, which is an alteration in the sequence of DNA (e.g. A is switched to T) that results in the construction of irregular proteins and leads to different physical results (e.g. different beak). Mutation occurs when DNA is damaged (by chemicals or X-rays) or when DNA polymerase (the DNA replication protein enzyme) makes a mistake in copying genes when attempting to replicate genes.
- Recombination — A second mechanism involves genetic information being swapped between chromosomes, generating hybrids. This is called recombination. This most commonly occurs when sperm meets egg — the single cell organism becomes a playpen for genes from the mom and dad. This explains why children look like their parents, but also have differences that make them unique.
- DNA: Repair — DNA also has built in codes for repairing itself. DNA can therefore build proteins that are specifically designed to help repair DNA from any harm. Mutations or problems in these repair genes can lead to the accumulation of DNA damage, which leads to more mutations, which can lead to something like cancer.
- Chapter Takeaway — DNA has built in functionality to create proteins that regulate, replicate, recombine, and repair itself. Regulation involves creating certain proteins that determine when genes are turned “on” and “off.”
Ch. 16: From Genes to Genesis
- Genes: Creating an Organism — One of the big questions that loomed over the field of genetics in the mid-1900s was: How can genes be orchestrated to create an organism out of a single cell? We all start as a single cell, so how do genes create the organism? How do genes know not to create feet where our hands should go, or to grow thumbs out of our noses? It’s a very complicated process, but the general idea is that genes organize the early embryo into main axis points (head vs. butt, front vs. back, left vs. right, etc.) using chemical gradients. Then a series of “segment” genes begin to divide the embryo into its major structural parts: brain, spinal cord, skeleton, skin, guts, etc. From there, organ-building genes begin constructing the organs, parts, and structures (limbs, fingers, eyes, kidneys, liver, and lungs). Throughout this process, genes are being turned on and off by proteins in order to create the organism as it should be built. Remember, all cells have the same exact genetic coding in them; proteins help activate and suppress different genes in each cell to create form and function in the body (e.g. developing feet where feet should be developed).
- Genes: Working Together — Although genes do act like a blueprint in assembling the body, they aren’t just a blueprint. They communicate and work closely with other genes and cells in the body to carry out their instructions and build the body. Without these relationships between genes and cells, the genome would be lifeless.
- Quote (P. 197): “The vast majority of genes, however, do not behave like blueprints. They do not specify the building of a single structure or part. Instead, they collaborate with cascades of other genes to enable a complex physiological function. These genes, Dawkins argues, are not like blueprints, but like recipes. In a recipe for a cake, for instance, it makes no sense to think that the sugar specifies the ‘top’, and the flour specifies the ‘bottom’; there is usually no one-to-one correspondence between an individual component of a recipe and one structure. A recipe provides instructions about process. A cake is a developmental consequence of sugar, butter, and flour meeting each other in the right proportion, at the right temperature, and the right time. Human physiology, by analogy, is the developmental consequence of certain genes intersecting with other genes in the right sequence, in the right space. A gene is one line in a recipe that specifies an organism. The human genome is the recipe that specifies a human.”
- Chapter Takeaway — Genes and cells work together to build an organism. Most genes are not simply one-to-one methods of instruction. Instead, they communicate and coordinate with other cells and genes in the body in a really complex process of activation and suppression. Mutations are sort of like errors in the genetic code that can cause problems when certain genes are incorrectly suppressed or activated.
Ch. 17: "Crossing Over"
- How Viruses Work — Viruses have a simple structure: they are usually just a set of genes wrapped inside a protein coat. It’s a complicated process, but when a virus enters a cell, it sheds its coat and begins to use the cell as a factory for copying its genes and manufacturing new coats, which results in millions of new viruses leaking out of the cell.
- Recombinant DNA: Creating Gene Hybrids — In the 1960s and 1970s, scientists began experimenting with mixing and matching genes. Some tried to mix genes from a virus with genes from bacteria. Others worked on mixing genes from two different types of bacteria. Both methods involved a similar process: using DNA-cutting enzymes to cut genes from each bacteria, mixing them up in a test tube, adding another enzyme to join the ends of the DNA strands, then transferring the new gene hybrid into another organism (usually some kind of bacteria) via cell infusion. In 1973, Herbert Boyer and Stanley Cohen successfully accomplished this, generating a new species of bacteria by combining genes from two other bacteria then injecting the hybrid gene into a separate bacteria using cell infusion. The cells then multiplied (cloned) inside the new organism — this was one of the first successful cases of genetic engineering. The two processes were called Recombinant DNA (creating a gene hybrid) and gene cloning (producing millions of copies of the gene hybrid by injecting it into a bacteria).
- Chapter Takeaway — Genetic engineering took off in the 1960s and 1970s, particularly thanks to the work of Herbert Boyer and Stanley Cohen. These early experiments involved splicing DNA using DNA-cutting enzymes to form a new genetic species.
Ch. 18: The New Music
- Gene Reading — As some scientists were “writing” genes by mixing and matching gene fragments (previous chapter), others were trying to master gene sequencing in order to “read” genes. In 1971, Fred Sanger began to devise a gene sequencing technique and in 1977 was able to use this technique to reveal the full sequence of a simple virus. In the same year, other scientists discovered that many types of animal DNA are split into modules, not continuous stretches of information; this was a further development in the science of gene sequencing or “reading.” By 1980, major and significant developments were made in technologies that would enable genetic engineering and the manipulations of genes. These technologies revolutionized the field of genetics.
- Cloning & Sequencing Genes — In the 1970s and 1980s, scientists began to develop technology to manipulate, clone, and sequence genes. One of the ways they did this was by figuring out how to “read” DNA. They were able to study RNA in various cells of the body (e.g. white blood cells, liver cells, neurons, etc.) and understand which codes resulted in which genes. In this way, they were able to put together “libraries” of code for each cell, then compare the library to the library of other cells to understand how certain genes. They could then create genetic hybrids (recombinant DNA) and implant them into bacteria in order to clone millions of copies of the gene hybrid (gene cloning).
- Quote (P. 223): “A biologist could thus generate a catalog, or ‘library’ of all ‘active’ genes in a cell — akin to a library of books grouped by subject. There would be a library of genes for T cells and another for red blood cells, a library for neurons in the retina, for insulin-secreting cells of the pancreas, and so forth. By comparing libraries derived from two cells — a T cell and a pancreas cell, say — an immunologist could fish out genes that were active in one cell and not the other (e.g., insulin or the T cell receptor). Once identified, that gene could be amplified a millionfold in bacteria. The gene could be isolated and sequenced, its RNA and protein sequence determined, its regulatory regions identified; it could be mutated and inserted into a different cell to decipher the gene’s structure and function. In 1984, this technique was deployed to clone the T cell receptor — a landmark achievement in immunology.”
- Quote (P. 221): “In 1969, if a disease-linked gene was found in humans, scientists had no simple means to understand the nature of the mutation, no mechanism to compare the altered gene to normal form, and no obvious method to reconstruct the gene mutation in a different organism to study its function. By 1979, that same gene could be shuttled into bacteria, spliced into a viral vector, delivered into the genome of a mammalian cell, cloned, sequenced, and compared to the normal form. In December 1980, in recognition of these seminal advancements in genetic technologies, the Nobel Prize in Chemistry was awarded jointly to Fred Sanger, Walter Gilbert, and Paul Berg — the readers and writers of DNA.”
- Chapter Takeaway — In the 1970s and 1980s, scientists began to devise ways to read genes. The technologies and methods that were built during this time opened up all kinds of possibilities for gene editing and manipulation. By understanding how genes were sequenced, we were able to better compare certain DNA strands in various cells and mutated genes. This opened up the opportunity to begin developing ways of editing genes.
Ch. 19: Einsteins On the Beach
- Asilomar Conference — The Asilomar Conference was a historic three-day conference organized by Paul Berg, Maxine Singer, and other colleagues to discuss the potential biohazards and regulation of gene manipulation. It was held in February 1975 at a conference center at Asilomar State Beach in Northern California. A group of about 140 professionals (primarily biologists but also including lawyers and media members) participated in the conference to draw up voluntary guidelines to ensure the safety of recombinant DNA technology. This conference was historic because profound technologies and processes for recombinant DNA and gene cloning had recently been discovered, and there was real concern about the safety, biohazard risks, and regulation of these practices going forward. Asilomar allowed scientists to discuss these concerns and develop a plan of self-governance for the future.
- Quote (P. 234): “Recombinant DNA had pushed genetics from the realm of science into the realm of technology. Genes were not abstractions anymore. They could be liberated from the genomes of organisms where they had been trapped for millennia, shuttled between species, amplified, purified, extended, shortened, altered, remixed, mutated, mixed, matched, cut, pasted, edited; they were infinitely malleable to human intervention.”
- Asilomar Document — To solidify the self-governance of recombinant DNA among scientists, a document was written at Asilomar that ranked the biohazard risks of various genetically altered organisms and offered recommended containment facilities for each level. Inserting a cancer-causing gene into a human virus, for example, would require the greater level of containment and safety, while placing a frog gene into a bacterial cell would merit minimal containment. The paper also urged continuous review of genetic manipulation procedures. The proposal was unanimously accepted.
- Chapter Takeaway — The Asilomar Conference was a historic conference in which scientists discussed the risks and regulations around recombinant DNA and gene manipulation technologies. There were major biohazard concerns about these technologies, and it was critical that scientists around the world developed some kind of plan for self-governance.
Ch. 20: "Clone or Die"
- Genentech Is Born — In 1976, venture capitalist Robert Swanson met with Herb Boyer, one of the founding fathers of recombinant DNA, and expressed a desire to start a company that would use gene cloning techniques to make medicines. The two agreed to the idea, and Boyer suggested Genentech (a combination of Genetic Engineering Technology) as the company name. It stuck. Genentech was born. The first product Genentech tried to create was insulin, a hormone that, at the time, was still being produced from mashed-up cow and pig pancreases. It had never been synthesized in a lab before. Genentech took aim at it.
- Quote (P. 239): “The first potential product, at least, was obvious from the start: insulin. Despite many attempts to synthesize it using alternative methods, insulin was still being produced from mashed-up cow and pig innards, a pound of hormone from eight thousand pounds of pancreas — a near-medieval method that was inefficient, expensive, and outdated. If Boyer and Swanson could express insulin as a protein via gene manipulation in cells, it would be a landmark achievement for a new company.”
- About Insulin — Insulin is the hormone (and protein) released from the pancreas that regulates blood sugar. In 1921, Frederick Banting and Charles Best successfully isolated insulin for the first time by grounding up dozens of cow pancreases. In 1953, Fred Sanger figured out the amino acid chain sequence that created insulin. There are more notes about insulin in The Song of the Cell and The Body.
- Genentech: Synthesizing Insulin — Genentech was not the only lab trying to synthesize insulin for commercial use. There was a massive race to be the first to do it. What made insulin so tricky to synthesize was its structure — the protein for insulin is made of two separate chains of amino acids amounting to 51 amino acids in total. Many proteins in the body don’t have that many amino acids. Genentech’s approach was to construct insulin from scratch by stitching together bases of DNA (A added chemically to the G and the C, etc., etc.). In August 1978, Boyer and his team pulled it off: they became the first lab to create recombinant insulin. This made insulin easier to produce and sell to patients with diabetes.
- Quote (P. 244): “By May 1978, the Genentech team had synthesized the two chains of insulin in bacteria. By July, the scientists had purified the proteins out of the bacteria debris. In early August, they snipped off the attached bacterial proteins and isolated the two individual chains. Late at night on August 21, 1978, Goeddel joined the protein chains together in a test tube to create the first molecules of recombinant insulin.”
- Genentech: Patenting Insulin — Two weeks after they successfully created recombinant insulin, September 1978, Genentech applied for a patent. Rather than trying to patent insulin, they patented their process for creating recombinant DNA — a process that could be used to create many different proteins, not just insulin. More than four years after applying for it, the USPTO granted Genentech the patent. It soon became one of the most lucrative, and most hotly debated, patents in the history of technology.
- Hemophilia & Factor VIII Mutation — Prior to the mid-1980s, people with hemophilia, a condition resulting in almost nonstop bleeding caused by a mutation in the gene for blood clotting (factor VIII), were scared to death about going to the emergency room. One of two outcomes usually happened: they would have to be cut open, which would lead to massive blood loss due to their hemophilia, or they would get some kind of disease because they would be given injections of factor VIII to stop the bleeding, and these injections were distilled from thousands of liters of human blood, which usually contained some kind of contamination. Many hemophiliacs transmitted HIV from these injections, for example.
- Synthesizing Factor VIII — In 1983, Genentech set out to clone the factor VIII gene in order to help hemophiliacs and others. Just as they did with insulin, their goal was to create the factor VIII protein artificially using gene cloning. But this required a different approach since the factor VIII gene was made up of 2,350 amino acids compared to insulin’s 51. Stitching it together from scratch would be impossible. The scientists instead took the approach of pulling the gene out of human cells, spooning it out as if extracting a worm from soil. By summer of 1983, Genentech managed to clone the gene. From there, they inserted the gene into a plasmid, which acts a vehicle to carry the gene. The plasmid was then introduced into a hamster. The process was successful, and in 1984 Genentech announced that they had purified recombinant factor VIII. In 1987, after FDA approval, the medication was used for the first time to treat a hemophilia patient. It worked.
- How Genes Work: Introns — This has been touched on previously in the book, but summarizing here. The DNA code for most genes in the human genome are interrupted by stretches of DNA called introns, which are like garbled stuffers placed in between parts of a message. Recall that the bases of DNA (A, G, C, T) are sequenced together in various ways to create a gene (think of the end products as a word/message). Introns are the spaces and punctuation around the message. For example, rather than the word ‘genome’, the actual gene reads gen……… om…… e. The introns in human genes are often enormous, stretching across vast lengths of DNA, making it virtually impossible to clone a gene directly — the intron-containing gene is too long to fit into a bacterial plasmid, and plasmids are the vehicles that scientists use to carry a gene hybrid into bacteria or another organism for cloning. Technology was later invented to essentially cut the introns from the DNA code, allowing scientists to clone a gene after snipping off the introns. This made creating gene hybrids, and placing them into plasmids, much easier.
- Proteins & Drugs — Proteins are the hubs of the biological world. They are the enablers and disables and are crucial to so many functions in the body. All drugs, pharmaceutical or otherwise, achieve their effects by binding to a protein target and enabling or disabling certain receptors on the protein, essentially turning molecular switches on and off. To have any kind of effect, the drug has to bind to specific switches on the protein, but only to a specific set of switches. A drug that binds to everything and turns everything on and off is no different than poison. More on this in Buzzed.
- Quote (P. 250): “Nearly every drug works by binding to its target and enabling or disabling it — turning molecular switches on or off. To be useful, a drug must bind to its switches — but to only a selected set of switches; an indiscriminate drug is no different from a poison. Most molecules can barely achieve this level of discrimination — but proteins have been designed explicitly for this purpose. Proteins, recall, are the hubs of the biological world. They are the enablers and the disablers, the machinators, the regulators, the gatekeepers, the operators, of cellular reactions. They are the switches that most drugs seek to turn on and off.”
- Proteins: Creating Medicine — Because proteins are so critical to our body’s functionality, they can be altered and manipulated in a lab, then inserted into the body via medicine, to achieve various results. The manufacturing of insulin and factor VIII to help diabetes patients and hemophiliacs, outlined above, are great examples of this. But to make a protein, you need its gene. Recall that proteins are simply the result of a chain of amino acids, which are created from RNA, which is simply a copy of a gene in DNA. This is why the development of recombinant DNA technology and processes in the 1970s and 1980s was so revolutionary. The cloning of human genes allowed scientists to manufacture proteins — and the synthesis of proteins opened opportunities to produce millions of biochemical reactions in the body.
- Quote (P. 250): “But to make a protein, one needs its gene — and here recombinant DNA technology provided the crucial missing stepping-stone. The cloning of human genes allowed scientists to manufacture proteins — and the synthesis of proteins opened the possibility of targeting the millions of biochemical reactions in the human body.”
- Genentech: Going Public — In 1980, Genentech sold 1 million of its shares in an IPO offering, listing itself on the stock market under the trading symbol GENE. Within a few hours, the company generated $35 million in capital. By then, pharmaceutical giant Eli Lilly had acquired a license to produce all of Genentech’s recombinant insulin. Sales at Eli Lilly rose from $8 million in 1983 to $90 million in 1996 to $700 million in 1998. Using its gene cloning technology, Genentech went on to create human growth hormone (HGH), a blood thinner to help heart attack and stroke victims, vaccines for hepatitis B, and more. In 1990, Genentech sold a majority stake for $2.1 billion. Swanson and Boyer became multimillionaires.
- Chapter Takeaway — Proteins are the hubs of the biological world, and have an impact on so many reactions in the body. The development of recombinant DNA technology and processes in the 1970s and 1980s opened up a lot of possibilities to generate gene hybrids and clone them to manufacture proteins that could be delivered into other organisms. The manufacturing of insulin and factor VIII offer great examples of this.
Ch. 21: The Miseries of My Father
- A Fall — In 2016, Mukherjee’s father had a bad fall. After several doctor’s visits and an MRI, it was determined that he had a condition called normal pressure hydrocephalus, which is thought to be a result of abnormal blood flow in the brain. Mukherjee learned everything possible about the condition and found that it is hereditary, a genetic disease. But there isn’t one single gene that causes the condition; it’s a result of several genes, and there are environmental cues that play a role as well.
Ch. 22: The Birth of a Clinic
- Victor McKusick: Cataloging Genes — “Genes make us human, and mutations make us different.” Acting on this belief, in the 1940s and 1950s, a scientist at John Hopkins named Victor McKusick decided to build a giant catalog of genetically-linked diseases in humans. By the mid-1980s, McKusick and his students had cataloged 2,239 genes linked with diseases in humans, and 3,700 diseases linked to single genetic mutations. By 1998, the catalog had grown to 12,000 gene variants (mutations) linked to traits and disorders. His catalog was sorted into three main buckets:
- Monogenic Diseases — A single gene or gene mutation leads to a specific disease. For the most part, no other genes or gene variants are involved. One Gene — One Disease.
- Polygenic Diseases (A) — Multiple genes and gene variants combine to cause a certain disease. With Down Syndrome, for example, children are born with an extra copy of chromosome 21, which has 300 genes strung on it. This extra chromosome, and the genes that come with it, cause the effects of Down Syndrome.
- Polygenic Diseases (B) — Once again, multiple genes contribute to a certain illness. Unlike some of the rare illnesses and syndromes that occur in the first two categories above, this category includes more familiar chronic illnesses, like diabetes, coronary artery disease, hypertension, depression, schizophrenia, infertility, and obesity. Many, many genes are involved in these illnesses. Hypertension, for example, comes in thousands of varieties and is under the influence of hundreds of genes, each contributing certain effects on blood pressure and the strength of blood vessels. With these polygenic illnesses, the effect of any single gene is dulled and environmental variables — diet, age, smoking, nutrition, exercise, etc. — play a bigger role than they do with the syndromes in the first two buckets.
- Victor McKusick: Findings From Catalog — There were four big takeaways from Victor McKusick’s catalog of genetically-linked diseases. A few of these helped clear up some of the misconceptions at the time about genes and their impact on diseases.
- Single Gene Diversification — Mutations in a single gene can cause a wide variety of diseases in different organs. In Marfan syndrome, for example, a mutation in a certain structural protein affects all connective tissue — tendons, cartilage, bones, and ligaments. The same structural protein supports large arteries and valves of the heart, so a mutation of this gene causes all kinds of diverse issues. In other words, one gene can sometimes affect multiple areas of the body.
- Multiple Genes Affect Physiology — The second finding was the converse of the first finding: multiple genes can influence a single aspect of the body. Hypertension is another good example of this. Many different genes contribute to blood pressure. It’s true that high blood pressure is often a genetic illness, but it’s also true that there is no “single gene” for hypertension.
- Environment Matters — For some diseases, genes are not the end-all-be-all; environmental cues can have a big impact on the expressivity of a gene. An example is the BRCA1 gene: this gene dramatically increases the chances of getting breast cancer, but some women with the gene do not get the cancer, and different mutations of the gene have different levels of effects.
- Mutations Are Just Variations — At the end of the day, mutations are simply variations of genes. They are deviations from the norm. A tall person in a nation of dwarves is a mutant in just the same way a child with Down Syndrome is a mutant among “normal” kids. Mutations are variants in DNA.
- Roe v. Wade — Roe v. Wade was a 1973 Supreme Court case that abolished most abortion laws in the United States. Norma McCorvey (who later switched her name to Jane Roe for the case) argued that she had a right to an abortion. Henry Wade was the defendant for the State of Texas. In a historic decision, the Supreme Court ruled in Roe’s favor. The Court also ruled that women had the right to an abortion decision in the two trimesters of pregnancy, but as the fetus develops its parenthood becomes more protected by the state and abortion could be restricted. This ruling led to a wave of prenatal genetic testing and subsequent abortions in the 1970s. If couples went through prenatal testing and found some sort of genetic issue, many decided to terminate the pregnancy. Between 1971-1977, the incidence of Down Syndrome, one of the illnesses easily detected by prenatal testing, fell by 20-40%.
- Chapter Takeaway — Some illnesses are caused by a single gene or gene mutation, while others are the result of many genes and gene mutations. It’s usually not as simple as one gene = one disease. The relationship between genotypes and phenotypes is often very tricky and complicated.
Ch. 23: "Interfere, Interfere, Interfere"
- Newgenics — The number of terminated pregnancies that arose from the Roe v. Wade Supreme Court ruling inspired a period of “newgenics” where people were making decisions about abortion based on prenatal genetic testing. This process of terminating pregnancies based on the presence of “inferior” genetics resembled the eugenics movement of the 1930s and 1940s that Hitler had taken to the extreme in Nazi Germany. Like the eugenics movement, “newgenics” was flawed in the sense that one gene doesn’t guarantee one result (phenotype). There are many other genes and environmental factors that contribute to the presence of an illness and the severity of that illness. It’s not a simple thing.
Ch. 24: A Village of Dancers, an Atlas of Moles
- Mapping Genes — The human genome is enormous. Finding certain genes within it can be like trying to pinpoint a needle in a haystack. To counter this problem, scientists in the 1970s began trying to find certain genes for certain illnesses by looking for links. This is because all genes are linked to each other on chromosomes. If you can find two traits that are linked, and the genetic location of one of those traits is known, you can use that to your advantage to find the set of unknown genes causing the other trait. For example, scientists were trying to find the genes for a disease called hemochromatosis, a hereditary illness that causes the body to absorb budge amounts of iron. They had discovered that the hemochromatosis gene was genetically linked to a certain immune-response gene. Prior work had mapped the immune-response gene to chromosome six — therefore the hemochromatosis gene had to be located on that chromosome.
- Quote (P. 281): “But as Botstein listened to Kravitz that morning in Utah, he was struck by a compelling idea: if such variant genetic signposts existed in the human genome, then by linking a genetic trait to one such variant, any gene could be mapped to an approximate chromosomal location. . . The polymorphisms would act like an internal GPS system for the genome; a gene’s location could be pinpointed by its association, or linkage, to one such variant.”
- Quote (P. 281): “The real key to human gene mapping, Botstein had realized, was not finding the gene, but finding the humans. If a large-enough family bearing a genetic trait — any trait — could be found, and if that trait could be correlated with any of the variant markers spread across the genome, then gene mapping would become a trivial task. If all the members of a family affected by cystic fibrosis inevitably ‘co-inherited’ some variant DNA marker, call it Variant-X, located on the tip of chromosome seven, then the cystic fibrosis gene had to sit in proximity to this location.”
- Mapping Genes: Huntington’s Disease — Scientists used this gene mapping technique to pinpoint the genetic code leading to Huntington’s disease, a terrible hereditary neurological illness in which people twitch and spasm involuntarily. Limbs dance randomly as if the person is a puppet being controlled by a puppet master. This illness typically does not appear until someone is 30 or 40 years old, and usually only one copy of the gene (from one of the two parents) is needed to get it. The late stages of this illness are marked by huge cognitive decline and complete loss of motor skills. Patients die of malnourishment (calories are burned while dancing), dementia, and infections. A team led by Nancy Wexler was able to take samples of blood from people in Venezuela (where the disease was prolific) and pinpoint a trait linked to Huntington’s disease. They then tracked that trait to chromosome four and searched the DNA strands one by one until they found the code that led to Huntington’s disease (1993). The code for Huntington’s disease creates a protein that is made of 3,144 amino acids, one of the largest proteins in the body.
- Mapping Genes: Cystic Fibrosis — In 1989, the gene mapping technique was also used to find the location in the human genome of the code that leads to cystic fibrosis, a terrible hereditary illness that affects the lungs, pancreas, and intestines. Cystic fibrosis affects the cells that produce mucus, sweat, and digestive juices. It causes these fluids to become thick and sticky. They then plug up tubes, ducts, and passageways. You need two copies of the mutated gene (one from each parent) to have any chance of getting this illness. Scientists used gene mapping techniques to find the gene that codes cystic fibrosis on chromosome seven. The most common mutation of this gene is one in which a single amino acid from the protein is deleted. This deletion creates a dysfunctional protein that is unable to move sodium chloride (salt) across membranes in the body, causing mucus and sweat to become thick and sticky. Once scientists found the gene, they cloned it in bacteria and sequenced it. They were then able to detect cystic fibrosis in prenatal genetic testing, which has led to a dramatic reduction of children diagnosed with it.
- Chapter Takeaway — In the 1980s and 1990s, scientists made huge strides in gene mapping. Certain gene mapping techniques allowed scientists to locate the genes for Huntington’s Disease and cystic fibrosis, among many others. Once located in the human genome, scientists were able to isolate, sequence, synthesize, and clone these genes, leading to the development of new medicines to treat some of these illnesses.
Ch. 25: "To Get the Genome"
- The Human Genome Project — In 1990, scientists from around the world, initially led by folks like James Watson (one of the two individuals who discovered the structure of DNA), initiated efforts to sequence the human genome, which contains more than 3 billion base pairs. The project was known as the Human Genome Project. The project was a massive undertaking; it took more than 10 years and led to the uncovering of all 20-25,000 human genes, making them accessible for further study.
- About Cancer — How does cancer work? It’s very complicated (Mukherjee has an entire book devoted to it!), but generally speaking cancers occur when the genes in a cell that control the cell’s growth become mutated and dysfunctional. In short, when these genes that control growth (there can be dozens of them) become disrupted via mutations, cells don’t know how to stop growing and eventually balloon into cancerous tumors. They can’t stop growing; the ‘on’ and ‘off’ switches that control the cell’s growth are hijacked. What’s so scary about cancer is that the issue involves our own “normal” cells turning against us. The goal of cancer research is to discover a way to give these growth genes within a cell their control back so they can regulate the cell’s growth correctly again.
- Quote (P. 296): “By the late 1970s, a full century after de Gouvêa, scientists began to converge on the uncomfortable realization that cancers arose from normal cells that had acquired mutations in growth-controlling genes. In normal cells, these genes act as powerful regulators of growth: hence a wound in the skin, having healed itself, typically stops healing and does not morph into a tumor (or in the language of genetics: genes tell the cells in a wound when to start growing, and when to stop). In cancer cells, geneticists realized, these pathways were somehow disrupted. Start genes were jammed on, and stop genes were flicked off; genes that altered metabolism and identity of a cell were corrupted, resulting in a cell that did not know how to stop growing.”
- Quote (P. 296): “The intimacy of the relationship between cancer genes and normal genes threw open a central challenge of cancer biology: How might the mutant genes be restored to their off or on states, while allowing normal growth to proceed unperturbed? This was — and still remains — the defining goal, the perennial fantasy, and the deepest conundrum, of cancer therapy.”
- Quote (P. 297): “In the late 1990s, at Johns Hopkins University, a cancer geneticist named Bert Vogelstein decided to create a comprehensive catalog of nearly all the genes implicated in human cancers. Vogelstein had already discovered that cancers arise from a step-by-step process involving the accumulation of dozens of mutations in a cell. Gene by gene, a cell slouches toward cancer —acquiring one, two, four, and then dozens of mutations that tip its physiology from controlled growth to dysregulated growth.”
- Cancer: Four Mechanisms of Acquisition — Normal cells in the human body can acquire these cancer-causing genetic mutations in four ways. All four lead to the same outcome: the inappropriate activation or inactivation of genetic pathways that control growth (stop and start buttons), causing dysfunctional cell division that defines cancer. The four mechanisms include:
- Environment — Things from the environment can cause cancer. Think smoking, exposure to UV light or X-rays, sun exposure, etc. These things attack DNA and change its chemical structure.
- Errors During Cell Division — Genetic mutations can arise during cell division (every time DNA is replicated in a cell, there’s a minor chance that the copying process generates an error — an A switched to a T, G, or C, for example).
- Inherited Genes — Mutant cancer genes in cells can also be caused by inherited genes from parents. Breast cancer, cystic fibrosis, and Huntington’s Disease are just a few of the many, many diseases that can be caused by inherited genes. Many cancers are related to inherited genes.
- Viruses — Mutated genes that cause cancer can be carried into cells via a virus of some sort. Viruses and bacteria have the unique ability to literally swap genes at any time. It would be like two friends swapping hair and eye color while hanging out — that’s what viruses and bacteria can do. As a result, some viruses could contain mutated genes that are cancerous. These can be a problem when they infect a person.
- Cancer: Extremely Diverse — A key feature of cancer is its incredible genetic diversity. This is one of the many reasons we’ve struggled to develop a cure for cancer. Two cancers with the same name are not alike — two people who have breast cancer likely have genetic mutations that are completely different. Cancer is incredibly diverse in its mutations.
- Quote (P. 297): “A fundamental feature of cancer was its enormous genetic diversity: two specimens of breast cancer, removed from two breasts of the same woman at the same time, might have vastly different spectra of mutations — and thereby behave differently, progress at different rates, and respond to different chemotherapies.“
- Chapter Takeaway — The Human Genome Project got underway in 1990. To generalize cancer — it occurs when the genes that regulate a cell’s growth become dysfunctional. The genetic mutations that lead to cancer lead to a situation where the cell doesn’t know when to stop growing. Once the genes are changed via these mutations, the cancerous cells replicate like crazy, eventually overtaking the body. There are four main ways the human body can acquire these cancer-causing genetic mutations.
Ch. 26: The Geographers
- Structure of Human Genome — The human genome contains more than 20,000 genes, but huge stretches of it don’t have genes. The enormous stretches of DNA between genes are called intergenic DNA and they are like long highways. In addition, genes themselves are broken into segments, with long spacers called introns jutted between protein-coding segments of the gene. Intergenic DNA and introns — the spacers and stuffers within genes — do not encode any protein information. Some of these stretches contain information to encode the on and off switches of certain genes. Other stretches have no known function. The structure of the human genome can thus be likened to a sentence that looks as follows. The words correspond to the genes, the ellipses correspond to the spacers and stuffers, and the occasional punctuation marks are the regulatory sequences of genes.

- The Worm Genome Project — As scientists were making progress on the Human Genome Project, on the side they were also working on decoding the human genome of a worm. In 1998, the Worm Genome Project was completed with the sequencing of the C. elegans worm. The worm genome marked the first complete sequencing of a multicellular organism. C.elegans was found to have 18,891 genes. In 2000, scientists completed the fruit fly genome. The fly was found to have 13,601 genes, 5,000 fewer than a worm.
- Human Genome Project Completed — In February 2001, scientists published their paper outlining the completed Human Genome Project. The report contained the complete sequencing of the human genome and its more than 20,000 genes and 3 billion DNA letters. It was a landmark moment in the history of man. We now had the human body’s instruction manual.
- Chapter Takeaway — The Human Genome Project was completed in the early 2000s. The project serves as the instruction manual of the human body.
Ch. 27: "Interfere, Interfere, Interfere"
- Facts About the Human Genome — After completing the Human Genome Project, our understanding of human genetics advanced quickly. What follows are a few quick facts about the human genome. We uncover more about it every year, but these are some interesting points that we’ve confirmed.
- Tons of Letters — The human genome has 3,088,286,401 letters of DNA (give or take a few). A recent estimate is about 3.2 billion letters. There are only four letters in this alphabet: A, G, C, T.
- Long Novel — Published as a book with a standard-size font, it would contain just four letters. AGCTTGAGGGG… and so on, stretching, page upon page, for over 1.5 million pages.
- Lots of Genes — It is divided into 23 pairs of chromosomes — 46 in total — in most cells in the body. It encodes about 20,687 genes in total — only 1,796 more than worms, 12,000 fewer than corn, and 25,000 fewer genes than rice or wheat. The difference between “human” and “breakfast cereal” is not a matter of gene numbers, but of the sophistication of gene networks Itis not what we have; it is how we use it.
- Gene Switchboard — It orchestrates the activation (on) or repression of (off) certain genes in only certain cells and at certain times, creating unique contexts and partners for each gene in time and space, and thus produces near-infinite functional variation out of its limited repertoire. This is how the various cells in our body know what to become and what to do. It’s why we don’t have hands growing where our nose is supposed to grow.
- Dynamic — It is incredibly dynamic and can reshuffle its own sequence to create variants of itself. Antibodies are a good example. These are missile-like proteins designed to attach themselves to an invading virus and help kill it. But viruses evolve constantly. To counter this, the genome can reshuffle sequences of DNA to create antibodies that match the pathogen. For example, s. . . tru. . . c. . . t. . . ure can be reshuffled to form an entirely new word: c. . . ome. . . t.
- Most of It Isn’t Genes — Surprisingly, genes comprise only a small fraction of the full genome. In fact 98% of it is dedicated to enormous stretches of DNA that are interspersed between genes (intergenic DNA) or within genes (introns). These act like highways. These long stretches don’t encode any RNA or proteins — they exist in the genome to either regulate gene expression (on and off switches) or for reasons we don’t get understand.
- Nonfunctional Genes — It contains thousands of genes that were once functional but have become nonfunctional. In other words, these genes don’t create RNA or proteins. The carcasses of these inactivated genes are littered throughout the genome, like fossils decaying on the beach.
- Variation But Similarity — It accommodates enough variation to make each one of us distinct, yet enough consistency to make each member of our species profoundly different from chimpanzees and bonobos, whose genomes are 96% identical to ours.
- The Genetic Process — The genetic code is simple: DNA is used to build RNA, and RNA is used to be build a protein. A triplet of bases in DNA specifies one amino acid in the protein.
- Chapter Takeaway — The human genome is fascinating. What’s maybe most surprising about it is that only a small portion of it is actually comprised of genes.
Ch. 28: "So We's the Same"
- Origin of Humans — Through human genome gene sequencing, scientists have concluded that the age of modern humans is about 100,000 years, which is really not very old in the grand scheme of evolution. Scientists have also traced back our origins to somewhere in sub-Saharan Africa. We then migrated north and east to populate the Middle East, Europe, Asia, and the Americas.
Ch. 29: The First Derivative of Identity
- X & Y Chromosomes: Determining Gender — Up until the early 1900s, the factors behind gender identity were relatively unknown. Nettie Stevens helped solved the mystery in 1903. While studying mealworms, Stevens discovered that a variation in just one chromosome correlated to a worm’s sex. Cells in male worms had two unpaired chromosomes, while female worms had chromosomes that all paired up perfectly. In the male worm, one of the unpaired chromosomes was larger in size, while the other was shorter and stubbier. Stevens called the shorter chromosome the sex chromosome. Why? In short, Stevens found that when sperm is created, it is made in two forms: one where chromosomes are short and stubby and one where chromosomes are normal. Whichever form of sperm reaches the egg first will determine gender.
- Quote (P. 358): “Cells from female worms inevitably possessed ten matched pairs. Cells from male worms, in contrast, had two unpaired chromosomes — a small, nublike band and a larger chromosome. Stevens suggested that the presence of the small chromosome was sufficient to determine sex. She termed it the sex chromosome. To Stevens, this suggested a simple theory of sex determination. When sperm was created in the male gonad, it was made in two forms — one bearing the nublike male chromosome, and another bearing the normal-size female chromosome — in roughly equal ratios. When sperm bearing the male chromosome — i.e., ‘male sperm’ —fertilized the egg, the embryo was born male. When ‘female sperm’ fertilized an egg, the result was a female embryo.”
- X & Y Chromosome Continued — Edmund Wilson later came along and called the short chromosome the ‘Y’ chromosome (male) and the normal chromosome the ‘X’ chromosome (female). Females obviously have an X chromosome already loaded into the egg. Whichever form of sperm from the male fertilizes the egg therefore determines the gender of the offspring. If the stubby Y chromosome sperm reaches the egg first, you’ll get an embryo with an XY chromosome, which leads to a boy. If the normal chromosome sperm reaches the egg first, you’ll get an embryo with an XX chromosome, which leads to a girl.
- Quote (P. 358): “Stevens’s work was corroborated by that of her close collaborator, the cell biologist Edmund Wilson, who simplified Stevens’s terminology, calling the male chromosome Y, and the female X. In chromosomal terms, male cells were XY, and females were XX. The egg contains a single X chromosome, Wilson reasoned. When a sperm carrying a Y chromosome fertilizes an egg, it results in an XY combination, and maleness is determined. When a sperm carrying an X chromosome meets a female egg, the result is XX, which determines femaleness.”
- SRY: The Gender Gene — We’ve established that the Y chromosome is the male chromosome. Interestingly, within the Y chromosome resides a single gene that completely determines gender. In 1989, scientists pinpointed the gene — a gene called SRY. When this gene is ‘on’, which it is 99% of the time on the Y chromosome, it produces male features in the person. When this gene is ‘off’ due to some mutation of the gene, which is rare but does happen, it creates a person who is a technically a man based on his XY chromosomes but develops female features. The person has all of the physical features of a woman, but he’s technically a man based on his chromosomes. This rare condition is called Shyer Syndrome.
- Chapter Takeaway — There is one gene, located in the Y chromosome, that determines gender. When this gene is ‘off’ it can produce female features in a person who is a technically a man based on his chromosome orientation.
Ch. 30: The Last Mile
- Genes Affect Normal Living — Most people only point to the impact of genes when dealing with an illness or addiction; something unusual happening in their life. In reality, genes influence both illness and normalcy. Our physiology, gender, sexual preference, temperament, allergies, personality, triggers, anxiety, and much more are heavily influenced by our genes. Variations in our genes cause slight differences in all of these areas, and that is why everybody is completely unique to themselves.
- Quote (P. 387): “Gender. Sexual preference. Temperament. Personality. Impulsivity. Anxiety. Choice. One by one, the most mystical realms of human experience have become progressively encircled by genes. Aspects of behavior relegated largely or even exclusively to cultures, choices, and environments, or to the unique constructions of self and identity, have turned out to be surprisingly influenced by genes. But the real surprise, perhaps, is that we should be surprised at all. If we accept that variations in genes can influence diffuse aspects of human pathology [diseases], then we can hardly be astonished that variations in genes can also influence equally diffuse aspects of normalcy.”
- Genes Provide Structure, Not Fate — Genes, mixed together from mother and father, help build an organism by increasing the probability that certain things will develop a certain way, but there is more to life than genes. For example, a set of genes in your genome may predispose you to a particular configuration of a nose, but the precise shape or length of the nose you develop remains unknowable. This applies to all areas of your personality as well. In short, genes help build an organism through probabilities, but they do not fully determine what you will become or accomplish in life. In this way, genes and the environment each play big roles. This is why identical twins (with the same exact genome) can go on to live incredibly different lives.
- Quote (P. 387): “The variations that you inherit from your parents, mixed and matched, specify variations in cellular and developmental processes that ultimately result in variations in physiological states. If these variations affect master-regulatory genes at the tip of a hierarchy, the effect can be binary and strong (male versus female; short statured versus normal). More commonly, the variant/mutant genes lie in lower rungs of cascades of information and can only cause alterations in propensities. Often, dozens of genes are required to create these propensities or predispositions. These propensities intersect with diverse environmental cues and chance to affect diverse outcomes — including variations in form, function, behavior, personality, temperament, identity, and fate. Mostly, they do so only in a probabilistic sense — i.e., only by altering weights and balances, by shifting likelihoods, by making certain outcomes more or less probable. Yet these shifts in likelihoods are sufficient to make us observably different.”
- Quote (P. 388): “Genes can describe the form or fate of a complex organism in likelihoods and probabilities — but they cannot accurately describe the form or fate itself. A particular combination of genes (a genotype) might predispose you to a particular configuration of a nose or personality — but the precise shape or length of the nose that you acquire remains unknowable. A predisposition cannot be confused with the disposition itself: one is a statistical probability; the other, a concrete reality. It is as if genetics can nearly beat its way to the door of human form, identity, or behavior — but it cannot traverse the final mile.”
- Chapter Takeaway — Genes have a major influence on your life, but they do not fully determine what you will become and accomplish. They may make things more or less likely to happen (e.g. addiction), but they are not the end-all-be-all.
Ch. 31: The Hunger Winter
- Nuclear Transfer — In 2012, John Gurdon was awarded the Nobel Prize for his experiments on nuclear transfer and cloning. These experiments involved taking the nucleus from one of an adult frog’s intestinal cells and transferring it into an empty egg cell. Although his success rate was abysmal, this “nuclear transfer” process occasionally birthed tadpoles with the same genome as the frog, and he was able to use this process to create the same tadpole (clones) over and over. Gurdon’s process was later expanded to other animals and led to the famous cloning of Dolly the sheep.
- Epigenetics: Chemical Stamps on Genes — Epigenetics is a hotly debated field within genetics that posits that cells in various areas of the body somehow place a permanent chemical stamp on the DNA in the chromosome of the cell in order to lock the cell into place. The actual genes are not altered; rather these chemical stamps (called methyl tags and histones) are sort of like notes, underlines, and bolded text that surround the genetic code in the cell and add context. Recall that every single cell in the body has the exact same genome (genetic coding) stored in its nucleus. These epigenetic stamps or “notes” help regulate gene expression by assisting other “master-regulator” proteins in the process of flicking genes on and off within the cell. This process of flicking genes on and off is what allows a red blood cell to understand its form and function. It’s what allows our liver cells to understand their form and function. It’s what allows our feet to grow where they’re supposed to grow. If all 20,000+ of our genes were expressed in every single cell, we would be a blob. Epigenetics also help explain why environmental factors matter; these notes and stamps are often appended to our genome after things happen to us. This explains why the epigenomes of identical twins become quite different over time.
- Quote (P. 401): “These [epigenetic] marks may function like notes written above a sentence, or like marginalia recorded in a book — pencil lines, underlined words, scratch marks, crossed-out letters, subscripts, and endnotes — that modify the context of the genome without changing the actual words. Every cell in an organism inherits the same book [genome], but by scratching out particular sentences and appending others, by ‘silencing’ and ‘activating’ particular words, by emphasizing certain phrases, each cell could potentially write a unique novel from the same basic script. . . The boldface and capitalized letters and the underlined words are epigenetic marks appended to the genome to impose a final layer of meaning.”
- Quote (P. 403): “If you sequence the genomes of a pair of identical twins every decade for fifty years, you get the same sequence over and over again. But if you sequence the epigenomes of a pair of twins over the course of several decades, you find substantial differences: the pattern of methyl groups attached to the genomes of blood cells or neurons is virtually identical between the twins at the start of the experiment, begins to diverge slowly over the first decade, and becomes substantially different over fifty years.”
- Genes & Cells: How They Interact — The passage below is a beautiful and simple way of understanding how genes (the genome and epigenetics) and cells work together to create a human. Ultimately, genes are nothing without cells; cells are the housing compartments that bring genes to life and allow for signaling and everything else. We start as a single-celled embryo and blossom into a 30+ trillion cell organism. The mechanisms behind that process are very complex, but the passage below provides a simple look at how the interaction between genes and cells makes it happen:
- Quote (P. 407): “Seconds after fertilization, a quickening begins in the embryo. Proteins reach into the nucleus of the cell and start flicking genetic switches on and off. A dormant spaceship comes to life. Genes are activated and repressed, and these genes, in turn, encode yet other proteins that activate and repress other genes. A single cell divides to form two, then four, and eight cells. An entire layer of cells forms, then hollows out into the outer skin of a ball. Genes that coordinate metabolism, motility, cell fate, and identity fire ‘on’. The boiler room warms up. The lights flicker on in the corridors. The intercom crackles alive. Now a second layer of information — instigated by master-regulator proteins — stirs to life to ensure that gene expression is locked into place in each cell, enabling each cell to acquire and fix an identity. Chemical marks [epigenetics] are selectively added to certain genes and erased from others, modulating the expression of the genes in that cell alone. Methyl groups are inserted and erased, and histones are modified. The embryo unfurls step by step. Primordial segments appear, and cells take their positions along various parts of the embryo. New genes are activated that command subroutines to grow limbs and organs, and more chemical marks are appended on the genomes of individual cells. Cells are added to create organs and structures — forelegs, hind legs, muscles, kidneys, bones, eyes. Some cells die a programmed death. Genes that maintain function, metabolism, and repair are turned on. An organism emerges from a [single] cell.”
- Chapter Takeaway — Epigenetics posits that there are chemical “stamps” and “notes” appended to our genetic code that add context to our genome. These stamps and notes don’t change our genes in any way, but they team up with various regulator proteins to affect gene expression (the switching on and off of genes in certain cells). The specifics around epigenetics are still being researched, and the idea is a hotly debated one.
Ch. 32: The Hunger Winter
- Gene Therapy — In the 1990s, the first gene therapy treatments were administered in patients with various genetic illnesses. The very first of these initial treatments helped children with a deficiency in their ADA gene and involved withdrawing 200 million T cells (and therefore, genes) from the patient via the blood, mixing the patient’s blood/cells/genes with 200 million retroviruses (gene delivery vehicles) that contained modified genes to correct the issue, and inserting the mixed liquid back into the patient. In this case, the retrovirus with the modified genes transferred the correct genes to the patient’s cells in the lab, not the body. Once scientists were convinced that the mixed cells contained the correct genetic coding to resolve the issue, they then inserted the liquid back into the patient. A few years later, a gene therapy treatment for a different issue ended with a dead patient. Rather than mixing the patient’s cells with the genetically modified virus in the lab, this treatment involved injecting the altered virus directly into the body, hoping that the virus would transfer the genes to cells in the body. It didn’t work; the patient’s body rebelled. The case put a lockdown on gene therapy for several years.
Ch. 33: Genetic Diagnosis — "Previvors"
- Breast Cancer: The BRCA1 Gene — In 1988, scientists pinpointed the location of a gene largely responsible for breast cancer in women — the BRCA1 gene. The gene is located on chromosome 17. This gene is almost single-handily responsible for breast cancer, and we’ve developed technology to be able to detect this gene in women before they exhibit symptoms. What’s interesting about this gene is that it may not lead to cancer at all. If you have it, there’s a chance (70-80%) you’ll get a deadly form of cancer and there’s a chance you won’t get cancer at all. Another interesting aspect of this gene is that the gene itself encodes a protein that is designed to repair damaged DNA strands. When the gene is mutated and dysfunctional, damaged DNA isn’t repaired properly, leading to further mutations and damage, eventually spiraling into breast cancer. More on how this gene works below:
- Quote (P. 441): “The [BRCA1] gene encodes a protein that plays a critical role in the repair of damaged DNA. For a cell, a broken DNA strand is a catastrophe in the making. It signals the loss of information — a crisis. Soon after DNA damage, the BRCA1 protein is recruited to the broken edges to repair the gap. In patients with the normal gene, the protein launches a chain reaction, recruiting dozens of proteins to the knife edge of the broken gene to swiftly plug the breach. In patients with the mutated gene, however, the mutant BRCA1 is not appropriately recruited, and the breaks are not repaired. The mutation thus permits more mutations — like fire fueling fire — until the growth-regulatory and metabolic controls on the cell are snapped, ultimately leading to breast cancer.”
- Schizophrenia — In 1908, psychiatrist Eugen Blueler introduced the term schizophrenia to describe patients with extreme cognitive disintegration. He described the condition as the brain “splitting,” leading to the name schizophrenia (derived from “split brain”). We know that there are at least 108 genes associated with schizophrenia, although we only know the identity of a handful of these culprits. Unlike BRCA1 and breast cancer, there is no single gene driving schizophrenia. In 2016, scientists did pinpoint a gene called C4 that seems to contribute to schizophrenia by over-pruning synapses in the brain. When these synapses get over-pruned, it leads to cognitive dysfunction. Interestingly, prior to this study, the C4 gene was primarily thought to be part of the immune system; it encodes proteins that recognize and destroy viruses and bacteria. More on this below:
- Quote (P. 445): “The strongest, and most intriguing, gene linked to schizophrenia is a gene associated with the immune system. The gene, called C4, comes in two closely related forms, called C4A and C4B, which sit, cheek by jowl, next to each other on the genome. Both forms encode proteins that may be used to recognize, eliminate, and destroy viruses, bacteria, cell debris, and dead cells — but the striking link between these genes and schizophrenia remained a tantalizing mystery. In January 2016, a seminal study partly solved the puzzle. In the brain, nerve cells communicate with other nerve cells using specialized junctions or connections called synapses. These synapses are formed during the development of the brain, and their connectivity is the key to normal cognition — just as the connectivity of wires on a circuit-board is key to a computer’s function. During brain development, these synapses need to be pruned and reshaped, akin to the cutting and soldering of wires during the manufacture of a circuit-board. Astonishingly, the C4 protein, the molecule thought to recognize and eliminate dead cells, debris, and pathogens, is ‘repurposed’ and recruited to eliminate synapses — a process called synaptic pruning. In humans, synaptic pruning continues throughout childhood and into the third decade of adulthood — precisely the period of time that many symptoms of schizophrenia become manifest. In patients with schizophrenia, variations in the C4 genes increase the amount and activity of the C4A and C4B proteins, resulting in synapses that are ‘over-pruned’ during development.”
- Gene Reading — It will soon be possible to scan every fetal genome and get a look at all potential mutations and rank them in order of severity. Right now, it’s possible to do this but only with monogenic diseases like cystic fibrosis, Huntington’s disease, and sickle-cell anemia that are largely driven by one gene and have a near 100% chance of developing. But soon, as technology continues to advance, it will be possible to see any mutation in any gene in a fetus and assign probabilities of each mutation manifesting itself at some point in the individual’s life. From there, parents can decide how to approach the pregnancy.
- Quote (P. 455): “As we investigate genes and genomes more deftly, more comprehensively, and with more computational power, we should be able to ‘read’ the genome more thoroughly — at least in a probabilistic sense. Currently, only highly penetrant single-gene mutations (Tay-Sachs disease, cystic fibrosis, sickle-cell anemia), or alterations in entire chromosomes (Down syndrome), are used in genetic diagnosis in clinical settings. But there is no reason that the constraints on genetic diagnosis should be limited to diseases caused by mutations in single genes or chromosomes.”
- Quote (P. 455): “Computational algorithms could determine the probability of the development of heart disease or asthma or sexual orientation and assign a level of relative risk for various fates to each genome. The genome will thus be read not in absolutes, but in likelihoods — like a report card that does not contain grades but probabilities, or a résumé that does not list past experiences but future propensities.”
- Chapter Takeaway — We will soon be able to scan and sequence the genomes of any fetus and determine if they have any disease-causing genes or mutations and their chances of actually getting the disease when they’re older. This is remarkable technology that may cause some parents to terminate a pregnancy entirely.
Ch. 34: Genetic Diagnosis — "Previvors"
- Gene Therapy: Two Flavors — Gene therapy comes in two flavors. The first involves modifying the genome of a nonreproductive cell, like a blood, brain, or muscle cell. The genetic modification of these cells affects their function, but it does not alter the human genome for more than one generation. Genetic changes to these cells are not passed down to the human embryo. The second form of gene therapy is to modify a human genome so the change affects reproductive cells. Once a genomic change has been introduced into a sperm or egg — i.e., into the germ line of a human being-the change becomes self-propagating. The change is incorporated permanently into the human genome and transmitted from one generation to the next.
- Embryonic Stem Cells — Embryonic stem cells are cells derived from embryos that have the ability to produce an endless wave of various cell types in the body. They are the replenishers. They are key for the future of gene editing because if scientists can make genetic changes in embryonic stem cells and then transfer those cells into an embryo, the stem cells will produce a nearly unlimited supply of those edited cells in the body.
- Quote (P. 475): “Consider the following steps in sequence: (a) the derivation of a true human embryonic stem cell (capable of forming sperm and eggs); (b) a method to create reliable, intentional genetic modifications in that cell line; (c) the directed conversion of that gene-modified stem cell into human sperm and eggs; (d) the production of human embryos from these modified sperm and eggs by IVF… and you arrive, rather effortlessly, at genetically modified humans.”
- Gene Editing: Cas9 Protein — The previous chapter was about the advancements that have helped us read a person’s genome. This chapter is more about the advancements we’ve made toward being able to edit the “germ line” of a person’s genes (reproductive cells). In 2011, a new gene editing method was introduced that is changing the game. It involves a bacterial protein called Cas9. Basically, Cas9 is introduced into human cells and then “guided” to a specific part of a cell’s genome (via a strand of RNA in the cell) to make deliberate edits or additions. These edits are made by making a “cut” in the genome that disables the targeted gene. Bacteria use this system to chop up the genes of invading viruses in the body, thereby disabling the invader. Scientists are trying to use the system via Cas9 to make purposeful cuts and additions in the genome.
- Cas9: How It Works — To further understand how Cas9 works, it’s good to use an analogy. Imagine the human genome as a huge library. The books are written in an alphabet with four letters: A, C, G, and T — the four building-block chemicals of DNA. Reframed as a library of books, with about 250 words per page and 300 pages per book, we might think of ourselves — or rather the instructions to build, maintain, and repair ourselves — as written in about 80,000 books. Cas9, when combined with a piece of RNA to guide it, can be directed to make a deliberate change in the human genome. Sticking with the analogy, it can be used to find and erase one word in one sentence on one page in one volume of that 80,000-book library. It’s a search and destroy eraser.
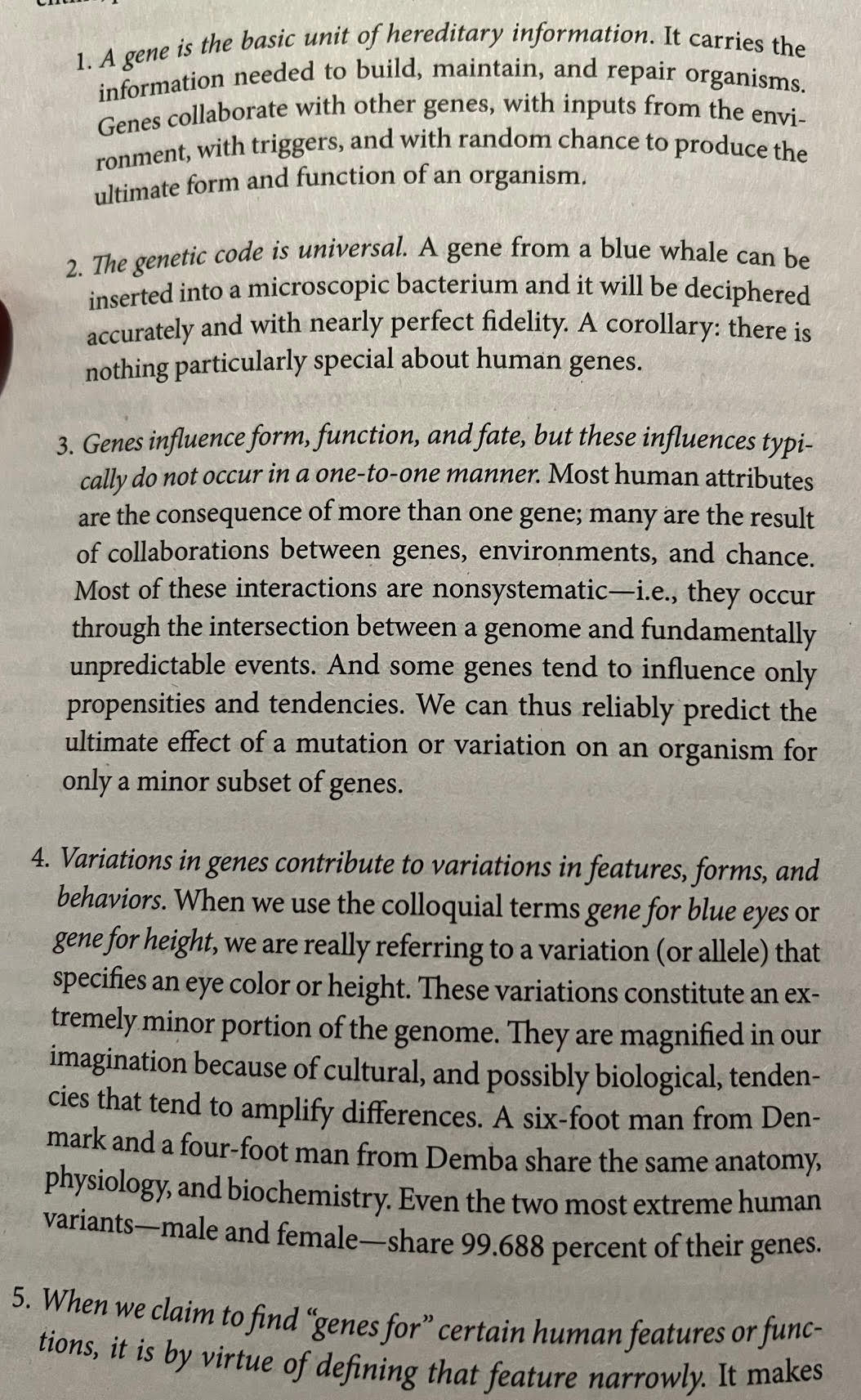
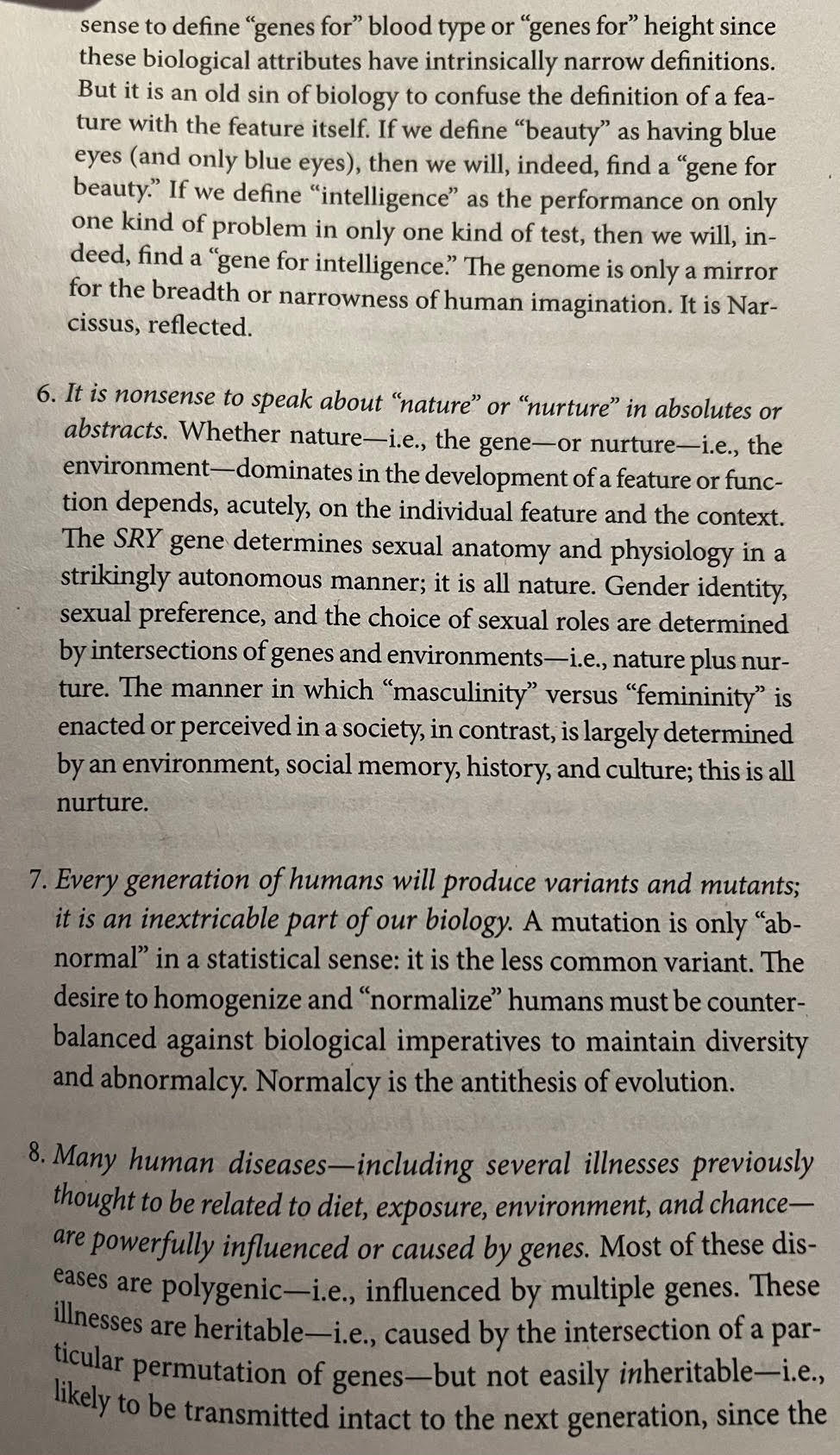
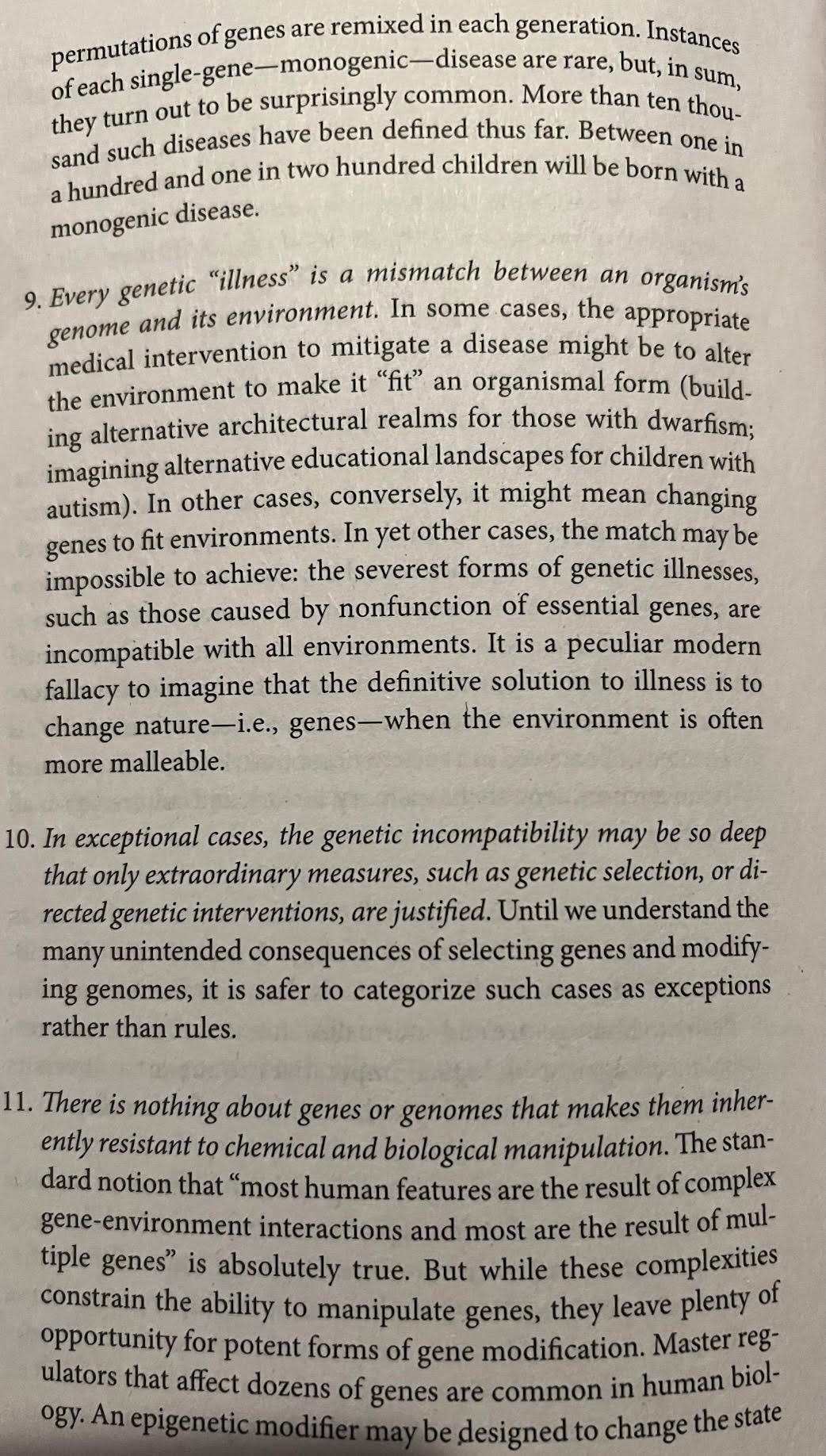
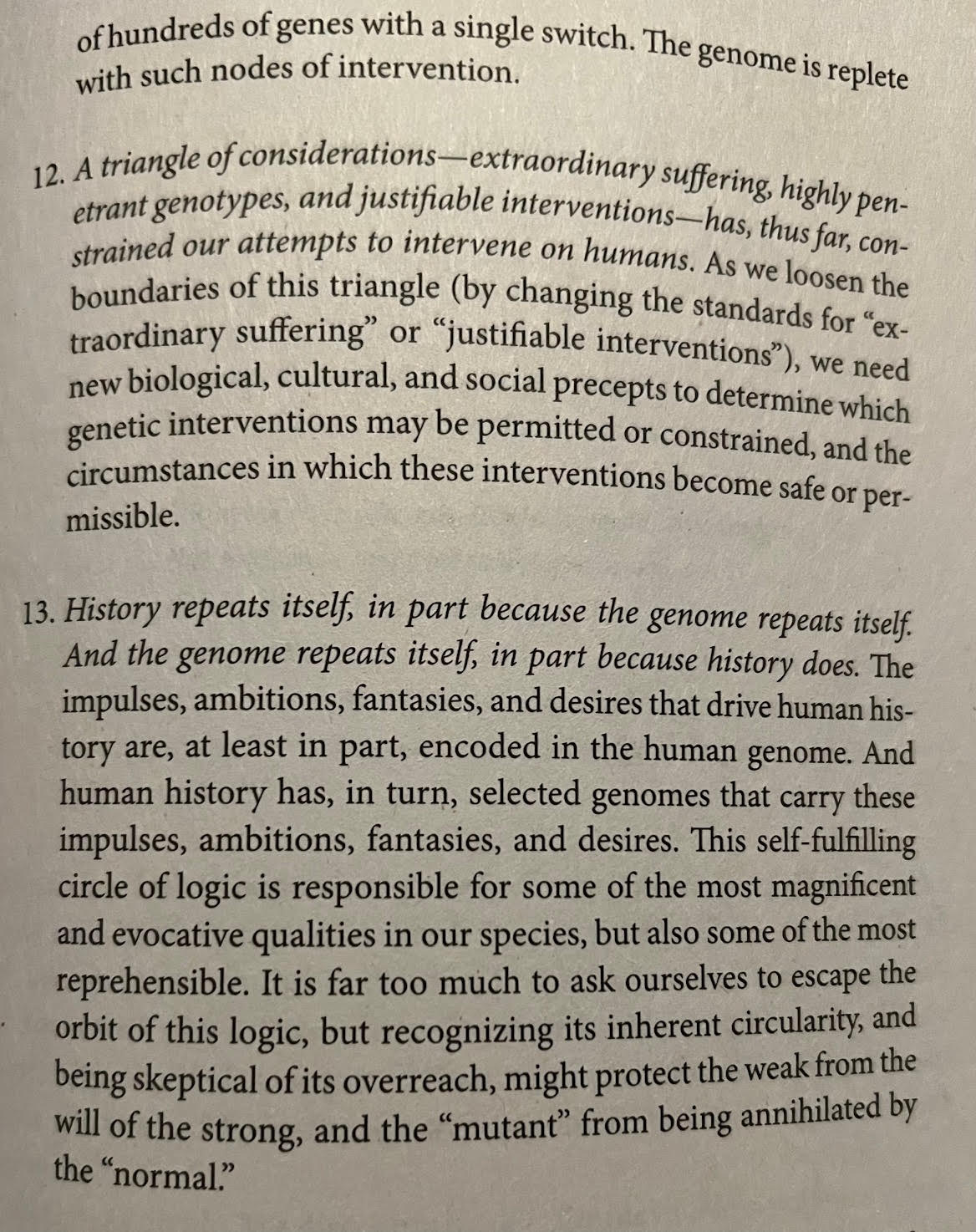
Epilogue
- The Future of Genetics: Previvors & Post-Humans — Moving forward, there’s a lot to be excited about in the field of genetics. We have the technology needed to manipulate genes and create better futures for people, both before people are even born and after they’re born. But there are a few challenges ahead that have to be solved:
- Learn More About the Genome — The Human Genome project was a major moment in the history of mankind, but there are still many questions we don’t have answers to regarding the human genome. Only a small slice of the human genome is actually made of genes; there are a lot of other things contained in the genome that we don’t fully understand. Also, much of our knowledge of genes and their functions comes from observing similar-looking genes in yeast, worms, flies, and mice. Learning more about human genes and deciphering the other random things in the genome will be important.
- Fate Maps — Once we have a strong command of all areas of the genome, we will be able to literally predict people’s futures, and not just regarding matters involving illness and disease. We will be able to predict (and potentially change) all kinds of things. We could even create “fate maps” based on gene sequencing.