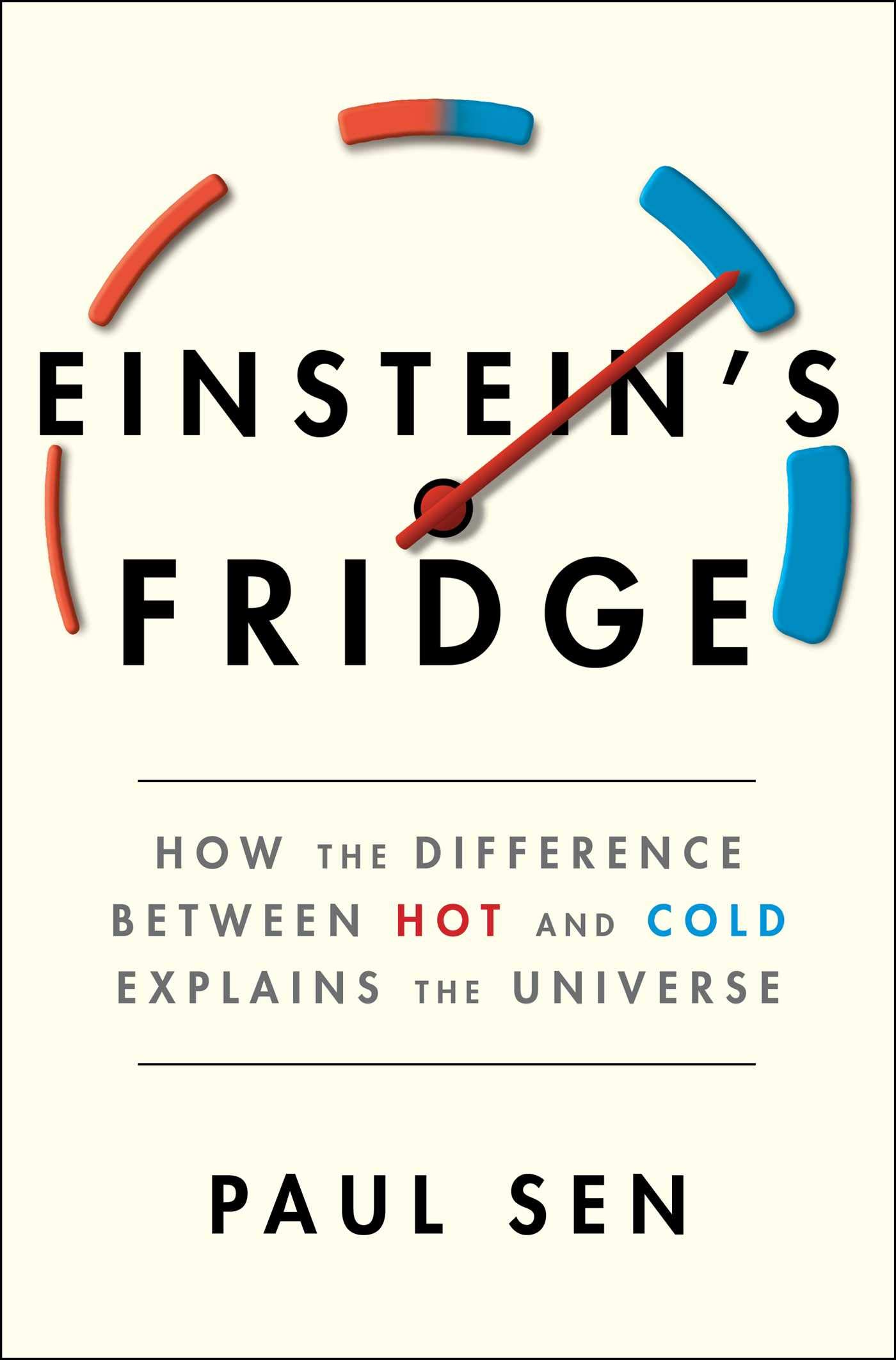
Short Summary
Paul Sen takes readers through a history of thermodynamics, reflecting on the findings of Sadi Carnot, Albert Einstein, James Joule, and more. Einstein’s Fridge tells the story of the scientists who harnessed the power of heat and ice and formulated a theory essential to comprehending our universe.
Key Takeaways
Kinetic Theory — When something is hot or cold, it’s an indication of how fast its particles are moving. Particles inside gases exert more pressure (i.e. move at higher speeds) when in an environment where the temperature is on the hot or warm side. When gases are in a cooler environment, the particles inside them have less pressure (i.e. move at slower speeds) and therefore feel cooler.
Entropy — Entropy is the second law of thermodynamics. It states that heat always flows from hot to cold. Heat can never flow from cold to hot naturally. This law is what led William Thomson to describe the ‘Heat Death of the Universe’ in 1852. The world will eventually run out of heat and die off, like anything else.
Refrigeration — Thermodynamics led to the discovery of the refrigerator, one of the most important devices in human progress ever created. Refrigerators force heat to pass from a cold interior to a warm exterior, essentially working against the second law of thermodynamics by slowing the process of entropy and decay.
Favorite Quote
“The kinetic theory of heat allows us to visualize what happens in the world we inhabit at scales too small to see — namely that everything around us consists of tiny particles in constant motion, and how hot or cold something feels is simply the way we, at a macroscopic scale, sense that motion.”
Book Notes 
Ch. 1: A Tour of Britain
- 19th Century Britain — Britain’s population, production, and economy boomed in the 19th century, much more than it ever had before. One of the big reasons was the number of steam engines. In the late 1700s, London had a handful of steam engines. By 1814, there were thousands.
- 19th Century France — The French economist Jean-Baptiste Say traveled to Britain and observed what was going on. France lagged behind Britain quite a bit, and Say put together a report in 1816 that convinced French engineers, businessmen, and politicians that the way to catch up was to exploit steam power.
- Coal — One of the issues France had was that their access to coal was a lot more scarce than Britain. It was therefore much more expensive for them to obtain it. This meant that French engineers cared a lot about efficiency and looked for ways to maximize the amount of useful work they could get out of coal. Additionally, the major universities around France focused on expanding on Newton’s laws (Newton was a student at Cambridge in Britain), while the students in London weren’t too focused on that.
Ch. 2: The Motive Power of Fire
- Sadi Carnot (1796-1832) — A French mechanical engineer known as, ‘the father of thermodynamics.’ In 1824, he published Reflections on the Motive Power of Fire, which explained that an engine works by moving heat from a hot reservoir to a cold one. To increase engine efficiency, one must increase the temperature difference. It’s the difference in temperature that creates the motor power, or work. Essentially, Carnot argued that without cold, heat is useless when it comes to creating power. This was revolutionary thinking at the time and had a big impact on steam engines. It also set the table for further study into thermodynamics.
- Quote (P. 19): “For any heat engine, the power it can produce from a given flow of heat is limited by the temperature difference between its furnace and sink. The only way to up this is to increase this temperature difference. Conversely, reducing the temperature difference will reduce the power output.”
- Quote (P. 20): “Internal combustion engines, jets, the giant turbines that generate electricity, and even the rockets that took humans to the moon, all are based on Carnot‘s discovery that the flow of heat from hot to cold is required to generate motive power.“
Ch. 3: The Creator's Fiat
- James Joule (1818-1889) — An English physicist and mathematician who studied battery-powered electric motors, which had been invented in 1830. Joule’s curiosity with battery-powered motors began while working at his family’s brewery in England. His father used steam engines, which burn expensive coal, and Joule felt he could find a better, cheaper way to produce power. Although he found that battery-powered motors would not be able to replace the steam engines at his family’s brewery, the findings he made while investigating were significant.
- Electricity and Heat — In 1840, Joule set up a lab and began experimenting with building batteries, electromagnets, and motors. One of his earliest observations was his most significant. He noticed that as an electric current runs through a wire, the wire becomes warmer. He therefore found that electricity can produce heat as well as do work by turning a motor. This finding ran against caloric theory, which stated that heat could not be produced or destroyed.
- Quote (P. 25): “Joule measured this effect with his hallmark diligence and deduced that whether or not caloric theory was true, there is a mathematical relationship between the heat produced, the magnitude of the current, and the resistance of the wire through which it flows.”
- Conservation of Energy: The First Law of Thermodynamics — Joule’s later experiments in the 1840s led to the first law of thermodynamics, which is today known as the conservation of energy. This law states that work (or motor power) can produce (or directly be converted into) heat. Prior to Joule’s findings, caloric theory was the widely accepted idea. Caloric theory stated that heat can’t be produced or destroyed.
Ch. 4: The Valley of the Clyde
- William Thomson (1824-1907) — An English physicist and mathematician who was one of the first to support Joule’s analysis of thermodynamics. Thomson was a science prodigy from birth. He studied math at Cambridge University in England, and later went to Paris to study under one of the premier physicists at the time, Victor Regnault. With Regnault, Thomson was able to observe how water and steam behaved when heated and cooled. It was in Paris where he encountered, and became obsessed with, the ideas of Sadi Carnot.
- Return to England — In 1845, Thomson returned to England, where at age 22 he became the professor of physics at Glasgow University, The leading educational establishment in the United Kingdom.
- Oxford, 1847 — It was in Oxford in 1847 where Thomson heard Joule’s speech on how work (or motor power) can create heat. Thomson was obsessed with Carnot’s ideas, which stated that heat was caloric and therefore could not be generated or destroyed. Although he believed Carnot’s analysis, Thomson believed Joule was on to something.
- ‘Thermodynamic’ — Thomson was the first to coin this term in his 1849 paper An Account of Carnot’s Theory of the Motive Power of Heat with Numerical Results Deduced from Regnault’s Experiments on Steam. In this paper, Thomson was able to prove that Carnot’s ideas about how the temperature change between hot and cold produces power were accurate. But he could not dismiss Joule’s ideas.
- Glacier Movement — Also in this paper and through his experiments to prove Carnot right, Thomson inadvertently explained how glaciers move.
- Quote (P. 39): “The pressure on the ice at the bottom of a glacier is so great that it melts, even though the temperature there is 0°C or lower. A layer of water is thus created under the glacier, allowing it to slide downhill. Remarkably, Sadi Carnot’s reflections on how steam engines work had led to an explanation for the movement of glaciers.”
- Glacier Movement — Also in this paper and through his experiments to prove Carnot right, Thomson inadvertently explained how glaciers move.
- The Missing Link — At this point in 1850, Thomson was able to prove Carnot’s ideas were correct; the temperature change between hot and cold is what produces power, or work. But he also believed Joule was right with his theories on heat — that work, or motor power, can create heat. Both Thomson and Joule believed there was a link, and they were determined to find some answers.
Ch. 5: The Principle Problem of Physics
- Hermann Helmholtz (1821-1894) — A German physicist and physician who was instrumental in furthering the idea of thermodynamics by studying how heat is generated in animals. Helmholtz grew up in Germany, a country that lagged behind England and France economically and in steam technology; in 1840, the capacity of fixed steam engines in the region’s factories were a mere 20,000 horsepower, far behind the 350,000 horsepower of Britain and the 34,000 horsepower in France. Helmholtz attended the Friedrich Wilhelm Institute in Berlin, where he studied medicine.
- Steam Advances — As Helmholtz was growing up, Germany was beginning to make advances in steam technology. In 1838, the first steam-powered train line in Prussia was established, running from Potsdam to Berlin. Helmholtz often rode the train, and was able to witness the power of steam.
- Vitalism — The idea that living organisms, in addition to the energy they received from food, water, air, etc., also possess a mysterious ‘vital’ life-giving force. While an organism was alive, this vital force controlled the physical and chemical processes that took place within it. This was a widely believed idea among many scientists at the time. Helmholtz opposed this ‘vitalist’ view and felt disproving it was a crucial step to putting biology on the same footing as physics and chemistry.
- On the Conservation of ‘Kraft’ — An 1847 paper written by Helmholtz in which he argued against vitalism and made the case that all heat released by an animal must come from chemical reactions that obey the same laws as those that govern the inanimate world. He also argued that whether it’s heat or electricity or movement, all these forms of ‘Kraft’ (or energy) can be turned into each other, but nothing is destroyed or created in the conversion.
- Heat — In the paper, Helmholtz also weighed in on the heat debate. He believed Carnot’s analysis that, given an unchanging quantity of heat, the temperature change between hot and cold generates work (or motor power), but he was also persuaded by Joule’s argument that mechanical work and electrical energy can turn into heat. In short, the debate on heat was still raging at this time.
Ch. 6: The Flow of Heat and the End of Time
- Rudolf Clausius (1822-1888) — A German physicist and mathematician considered one of the central founders of the science of thermodynamics. While at Berlin University, Clausius analyzed the work of Helmholtz, Carnot, Joule, and Thomson.
- Heat Debate — Clausius finally solved the problem that baffled his predecessors, and delivered his findings in a historic paper published in the Annals of Physics in 1850. In the paper, Clausius proved that both Carnot and Joule were correct in their arguments. His main finding was that heat can be both created and destroyed, and it must flow from hot to cold for work to be created.
- Heat and Engines — The flow of heat from hot to cold is what fires the pistons back and forth in an engine and produces motor power that can move cars, planes, etc. Clausius explained this process in his paper. In an engine, some heat flowing in from the furnace becomes work, some is discarded. As the sink’s temperature falls, this split becomes even more favorable. Then there comes a point when the sink is so cold that all the heat from the furnace becomes work. This is the end of the line. An engine can do no better than turn all the heat it receives into work. Otherwise it would be generating work from nothing, which would violate of the law of energy conservation.
- Takeaway — Essentially, engines have a furnace, where there’s heat, and a sink, where there’s cold. Engines are powered by the transfer of heat from the furnace to the sink — that’s what moves the pistons and generates motor power. The greater the difference in temperature between the furnace and sink, the more work/power is generated. When the two areas are relatively the same temperature, less work is produced.
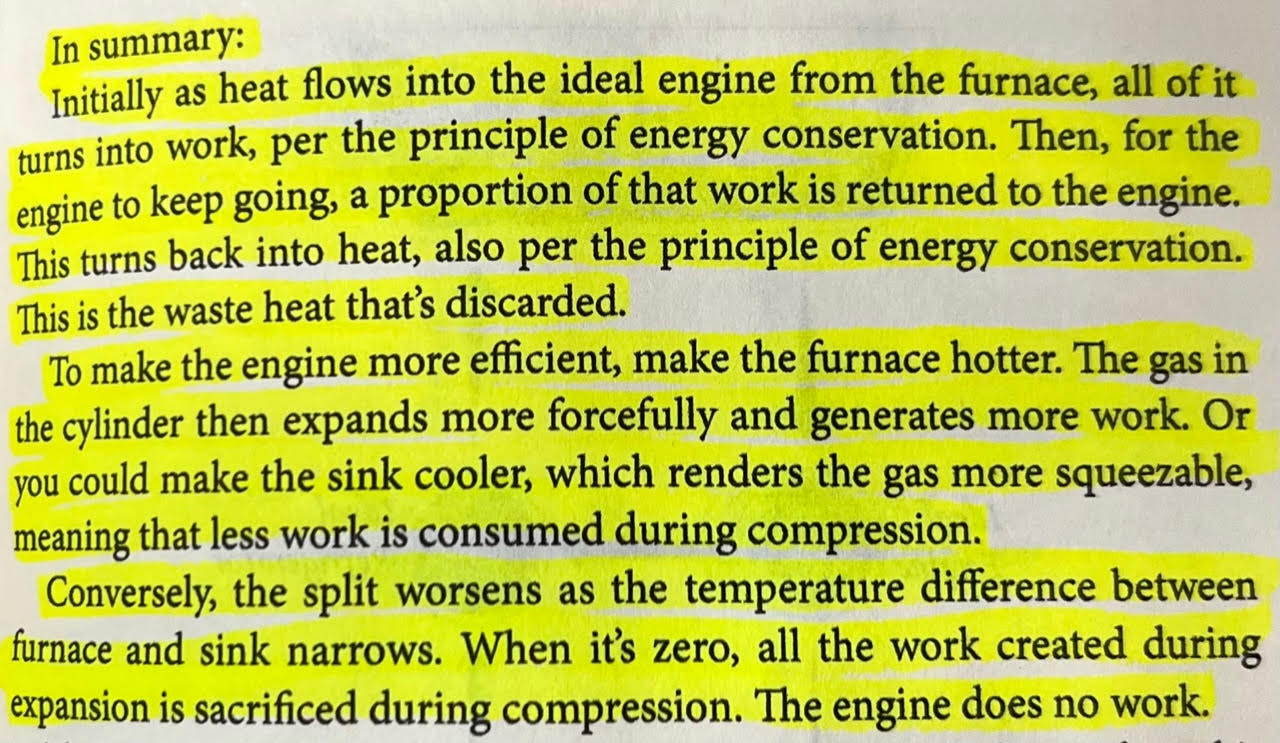
- Laws of Thermodynamics — Clausius’s paper essentially led to the discovery of the first two laws of thermodynamics. These are:
- First Law — Conservation of energy. One of the laws that governs our world in so many areas. Heat and work can be converted into each other as Joule had explained, but the total amount of heat plus work remains the same. So in a tight area, energy can only be transformed into another form of energy; more can’t be created and it cannot the energy cannot be destroyed. Essentially, the amount of energy before will always equal the amount of energy after.
- Second Law — This law is essentially the idea of entropy, which states that heat always flows from hot to cold. Heat never spontaneously flows from cold to hot. That’s not possible naturally.
- ‘Heat Death of the Universe’ — In 1852, Thomson published a paper in which he discussed the concept of irreversibility, the idea of a one-way dispersal of energy. Essentially, heat only flows from hot to cold, never the other way. That’s the second law of thermodynamics. This law led Thomson to conclude that the universe will eventually wind down and die after all of its heat flows from hot to cold and completely dissipates, an idea that became known as the ‘heat death of the universe.’ All big events that have happened in the cosmos was a result of heat dissipating.
- Iron Bar Metaphor — To explain his views, Thomson used the metaphor of an iron bar. When one side of the iron bar is hot, it flows to the other side of the bar and heats up the cool side. This transfer of heat from one side to the other can’t be reversed. The metaphor is true for life and the universe.
Ch. 7: Entropy
- Entropy — Clausius developed this idea in the 1850s. It essentially describes how heat flows and redistributes itself. It’s a measure of heat dispersion.
- Ex. House — Clausius used a house as an example of this idea of entropy. Imagine a large house with many rooms. Some have radiators and are hot, while some are cold. All of the doors are shut between the rooms. Now, turn off the radiators and open the doors to the rooms. Heat flows out of the warm rooms and into the cold ones. Soon every part of the house will have the same temperature. Entropy is considered high when the doors are open and heat is more evenly distributed throughout the house. Entropy is considered low when there are ‘pockets’ of heat, as seen in the hot and cold rooms when the doors were shut.
- Ex. Oven — Heat up a kitchen oven. Once it’s hot, turn off the power and open the oven door. Entropy says that heat always moves from hot to cold, so the temperature of the air inside the oven will cool until it’s the same as that of the room. The heat in the oven will essentially move outward and find the cool areas of the room. That’s entropy in motion.
- Quote (P. 68): “By defining entropy in this way, Clausius found a mathematical way of stating his law that heat always flows from hot to cold unless prevented from doing so.”
- More on Entropy — Clausius also found that the measure of entropy in a hot room falls less than the amount that entropy rises in a cold room. This is because you feel the difference more when heat enters a cold space than you do when heat leaves an already warm place.
- Noise Analogy — To understand this, imagine a loud bar right next to a quiet library. When five rowdy people leave the bar, the noise drop is fairly unnoticeable in the bar. But when those five rowdy people then enter the quiet library, the disruption and noise is very noticeable. It’s the same when heat flows into a cold room. That’s why the entropy rise in a cold room is greater than the entropy drop in a warm room when heat flows between the rooms.
Ch. 8: The Motion We Call Heat
- Daniel Bernoulli (1700-1782) — A Swiss mathematician and physicist remembered for his applications of mathematics to mechanics, especially fluid mechanics, and for his pioneering work in probability. He also contributed to the debate on heat through his creation of the Kinetic Theory of Gases. His findings helped set the table for later discoveries in the field of thermodynamics.
- Kinetic Theory of Gases — In 1738, Bernoulli wrote about Isaac Newton’s mechanics and how they applied to temperature change. His finding here was called Kinetic Theory, and was a landmark discovery. In essence, Bernoulli found that the particles inside gases exert more pressure (i.e. move at higher speeds) when in an environment where the temperature is on the hot or warm side. When gases are in a cooler environment, the particles inside them have less pressure (i.e. move at slower speeds) and therefore feel cooler.
- Quote (P. 77): “Bernoulli noted that heating a gas increases the pressure it exerts. With the balloon, if you heat it, the air inside will expand as it presses against its rubber walls with greater force. And if you take an inflated balloon and put it in a fridge to cool it down, you will see it shrink in size as the pressure exerted by the air inside it drops.”
- Quote (P. 78): “Bernoulli reasoned as follows: if heating a gas causes it to exert greater pressure, and if pressure is a direct result of the speed with which the air particles move, then adding heat to a gas must cause these particles to zip about a greater speed. In other words, hot air feels hotter than cool air because particles in hot air zip about at a much greater velocity than in cold air.”
- Takeaway — Kinetic Theory explains why air in the summer feels hotter than air in the winter. The air particles are moving faster on a hot day, so the air feels hotter on our skin. It also explains why a cup of hot chocolate feels hot — the molecules in it are moving at a higher velocity/speed/pressure.
- Kinetic Theory of Gases — In 1738, Bernoulli wrote about Isaac Newton’s mechanics and how they applied to temperature change. His finding here was called Kinetic Theory, and was a landmark discovery. In essence, Bernoulli found that the particles inside gases exert more pressure (i.e. move at higher speeds) when in an environment where the temperature is on the hot or warm side. When gases are in a cooler environment, the particles inside them have less pressure (i.e. move at slower speeds) and therefore feel cooler.
- Clausius and Kinetic Theory — In 1857, Clausius backed up and expanded on Bernoulli’s idea of Kinetic Theory in his paper The Nature of the Motion We Call Heat. In this paper, Clausius confirms Bernoulli’s hypothesis that temperature is dictated by how fast the particles in the gas are moving. Other huge ideas in this paper include:
- Solids, Liquids, and Gases — Clausius explained that all matter consists of trillions of particles in constant motion. In solids, these molecules vibrate around a fixed position. In liquids, the particles are in constant flux, making bonds and breaking them at the same rate to produce a fluid form. In gases, the molecules are free to move independently and in any direction.
- Evaporation — When you leave a bowl of water out on a warm day, a good amount of the water will evaporate over time. Clausius explained that the reason for this is that the particles on the surface of the liquid (water) are making and breaking bonds, but there are no particles to bond with above the surface. So, every now and then, a particle on the surface breaks free from a particle below and has nothing to attach to above. This means the particle will break free of the liquid and evaporate into the air.
- Why We Can Breathe — Clausius also explained why the earth’s atmosphere is able to retain so much of the gases oxygen and nitrogen. Isaac Newton had previously found that the earth’s escape velocity is 11 km/sec — anything traveling faster than this will escape earth’s gravity and be lost into space. Clausius found that the average speed of an oxygen molecule is 0.5 km/sec, which keeps it in the earth’s atmosphere and allows us to breathe.
- Gas Movement — Clausius touched on why gases don’t travel in a straight line. A criticism of his analysis from one of his colleagues was that you should be able to smell a gas with a lot of odor, like chlorine, instantly from the other side of the room when it’s released. But Clausius explained that the molecules in gases move like bumper cars; they don’t move in a straight line. When a gas molecule begins moving, it quickly bumps into another molecule, which deflects its path and causes delayed movement.
Ch. 9: Collisions
- James Clerk Maxwell (1831-1897) — A Scottish mathematician and scientist responsible for the classical theory of electromagnetic radiation. He was also known for confirming Bernoulli and Clausius’s findings regarding Kinetic Theory.
- Testing Kinetic Theory — Maxwell verified Kinetic Theory in 1860 by testing his own prediction that the viscosity (or stickiness) of gas is unaffected by its pressure. He created a contraption in his lab and found that the viscosity of the air remained the same throughout the entire range of pressures he tested. By doing so, he cemented Kinetic Theory.
- Quote (P. 93): “The kinetic theory of heat allows us to visualize what happens in the world we inhabit at scales too small to see — namely that everything around us consists of tiny particles in constant motion, and how hot or cold something feels is simply the way we, at a macroscopic scale, sense that motion.”
- Interesting Fact — Air is made up of nitrogen and oxygen molecules. Nitrogen molecules make up 78% of air around you and they are colliding into you all the time. At room temperature, the most likely speed at which a nitrogen molecule will strike you is around 420 meters per second. That’s over 900 mph.
- Interesting Fact — The coldest temperature recorded on earth is around -90°C at the Vostok Station in Antarctica. Here, the most likely molecular speed of a nitrogen molecule is down to about 740 mph. Again, according to Kinetic Theory, things that feel colder — air, food, drinks, etc. — are made up of molecules that are moving with less pressure or speed.
Ch. 10: Counting the Ways
- Ludwig Boltzmann (1844-1906) — An Austrian physicist and philosopher. Boltzmann used statistics to explain and solidify the second law of thermodynamics, which essentially states that the entropy of the universe always increases. His findings on the second law of thermodynamics were delivered in a paper he published in 1872.
- Confirming the Second Law of Thermodynamics — The second law of thermodynamics basically states that heat flows from hot to cold, which also sums up the idea of entropy. Boltzmann showed why this occurs. Essentially, the air in any room consists of a large number of particles that swap kinetic energy back and forth. Remember that the particles in warmer air are moving with more speed than particles in cooler air, so the kinetic energy in hot air is greater than the kinetic energy in cold air. Boltzmann showed that entropy/the second law of thermodynamics (essentially the redistribution of heat) occurs because molecules with greater kinetic energy collide with molecules containing less kinetic energy and a transfer of kinetic energy occurs until all molecules in the room have about the same kinetic energy and the temperature is therefore equaled out.
- Ex. Oven — When a kitchen oven is heated, turn off the power and open the oven door. What happens is entropy, or the second law of thermodynamics. Boltzmann’s point was that the molecules that make up the hot oven air have particles that are moving with greater speed and kinetic energy than the cooler air on the outside of the oven. When the oven door is opened, those hot air molecules collide with the cool air molecules and transfer their kinetic energy in the process until the warm oven air is completely redistributed into the cooler air and the temperature in the room evens out.
- Statistics — Boltzmann was unique because he used statistics to make his point on how the transfer of kinetic energy occurs. His idea was that there is a far greater statistical probability of having molecules arrange themselves evenly in a room than there is the probability of having a bunch of molecules with high kinetic energy form in a cluster in one spot in the room. So, when the restrictions that are sealing off a warm spot in a room or house are released, it’s statistically natural that the molecules with high kinetic energy (or heat) will blend with the molecules with less kinetic energy and redistribute to the cooler areas of the room.
- Josiah Willard Gibbs (1839-1903) — An American scientist whose work on thermodynamics was instrumental in transforming physical chemistry into a rigorous inductive science. Gibbs is known for showing how the laws of thermodynamics are applied to the material world. He wrote two papers around 1873 that were highly influential in the field of physics and thermodynamics.
- Refrigeration — Gibbs’s findings helped lead to the refrigerator, one of the biggest material examples of thermodynamics at work. The refrigerator has been one of the most important devices in human progress and well-being. By greatly extending the time for which food can be safely stored and transported, the refrigerator enabled the greatest improvement in human nutrition since our prehistoric ancestors discovered cooking.
- Quote (P. 110): “These devices force heat to pass from a cold interior to a warm exterior, which is the opposite direction to the one in which heat flows spontaneously. The purpose of this is to create a space where the relentless increase of entropy is slowed down. Although a refrigerator is a cool box, that’s a means to an end. Its ultimate purpose is to slow down decay and putrefaction, which are both examples of entropy increasing. Think of a refrigerator as a device inside which time slows down.”
- Takeaway — Refrigerators essentially slow down entropy and time. They allow food to have a longer life because they slow the process of the second law of thermodynamics.
- Interesting Fact — In the 1870s, the first ships with mechanical refrigeration began carrying frozen meat and poultry across the Atlantic.
- Quote (P. 110): “These devices force heat to pass from a cold interior to a warm exterior, which is the opposite direction to the one in which heat flows spontaneously. The purpose of this is to create a space where the relentless increase of entropy is slowed down. Although a refrigerator is a cool box, that’s a means to an end. Its ultimate purpose is to slow down decay and putrefaction, which are both examples of entropy increasing. Think of a refrigerator as a device inside which time slows down.”

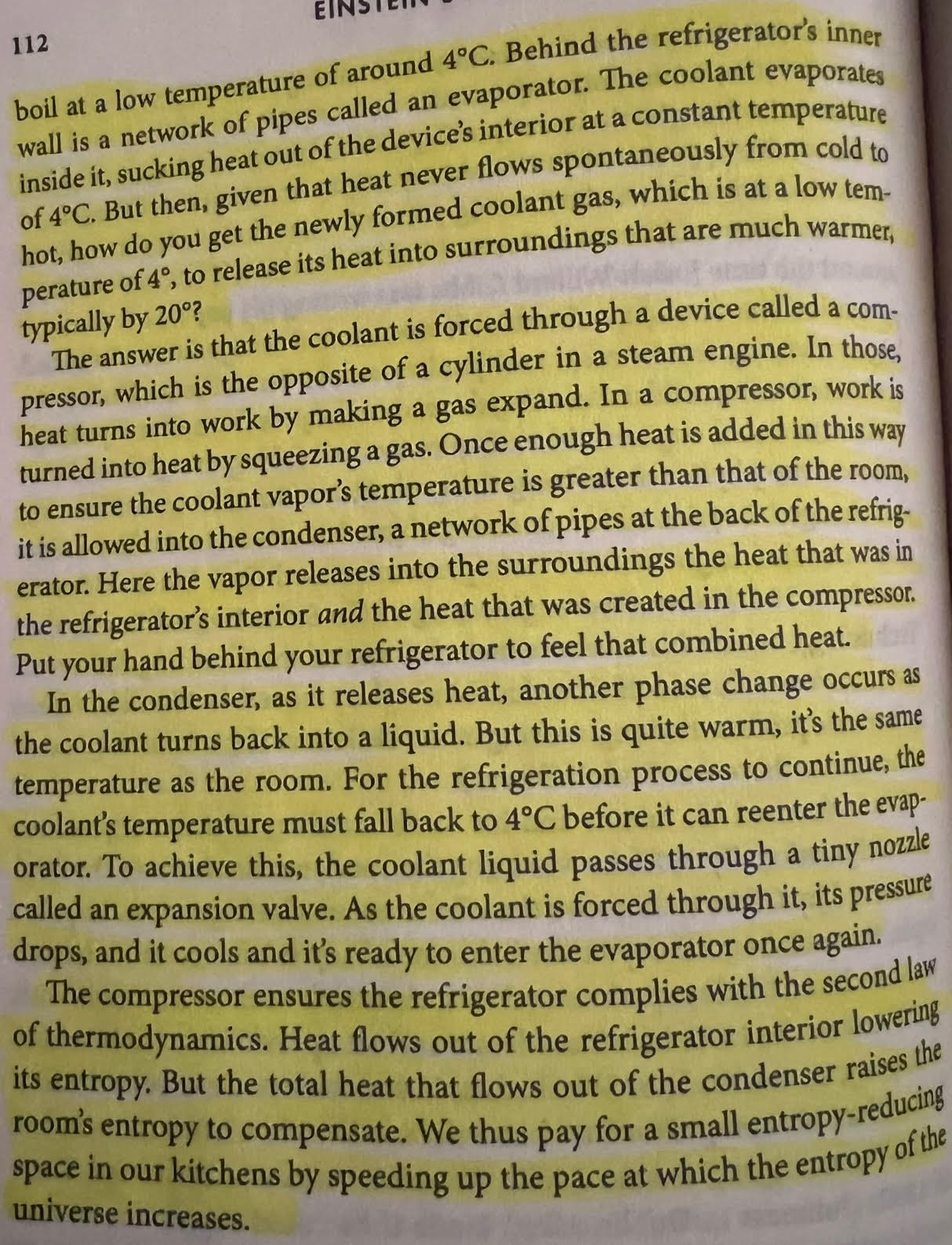
Ch. 11: The Terroristic Nimbus
- Gibbs’s Law — Gibbs successfully combined the first two laws of thermodynamics into one law that became known as Gibbs’s Law, which states that the flow of energy is the means by which the entropy of the universe is created.
- Cycle of Life — Essentially, this law is the cycle of life. Carbon dioxide breathed out by animals is absorbed by plants, which make food and oxygen and so on. The cycle needs a constant supply of Gibbs free energy to turn. Crucially, at each step of the cycle, a small amount of free energy is lost as heat. This means that at each step the entropy of the universe goes up. Overall, life is an effective way of increasing the entropy of the universe.
- Interesting Fact — Mach 1 is the name given to objects that travel faster than the speed of sound. The name is in reference to Ernst Mach, an Austrian physicist and philosopher. He took the first photographs of the shock waves created by objects that travel faster than the speed of sound.
- Top Gun — The jets flown in the military often reach Mach 1 and beyond that, as portrayed in Top Gun II when Tom Cruise was pushing Mach 9 in the opening scene.
Ch. 12: Boltzmann Brains
- Boltzmann Brains — Boltzmann’s studies about entropy and the statistical probabilities of atom collisions led him to believe that the universe was created via a large number of random fluctuations. This concept has been rejected by most modern cosmologists and astrologists.
Ch. 13: Quanta
- Heat Flow — There are three ways heat can flow out of an object. All three can be observed in most kitchens. These include:
- Conduction — This is how hot plates transfer heat. Think stove heating a pan. The heated surface of the plate is in contact with the underside of a pan, and the heat flows from one to the other.
- Ex. Saucepan — Kinetic theory explains this process. As the hot plate’s temperature rises, the molecules in it start moving at faster speeds. This sequence is transferred to the saucepan, which has molecules that then begin to vibrate at faster speeds. The result is that the saucepan, and its contents, rises in temperature.
- Convection — Occurs in ovens. Kinetic theory explains this as well. The heating elements within the oven’s wall cause the air molecules within it to zip about more quickly. These then collide with molecules deeper in the oven, increasing their speed, and soon the entire oven’s temperature rises.
- Radiation — This one is linked to light. Turn on a grill, and as the element’s temperature rises, it glows red. In addition to the visible red light, it’s also giving off infrared light, which is what feels hot. When this strikes an object, say the sausages in the grill pan, it causes their molecules to vibrate, raising their temperature.
- Conduction — This is how hot plates transfer heat. Think stove heating a pan. The heated surface of the plate is in contact with the underside of a pan, and the heat flows from one to the other.
- Max Planck (1858-1918) — A German theoretical physicist whose discovery of energy quanta won him the Nobel Prize in Physics in 1918. He discovered quanta in 1900. His discovery was so big that the pre-Planck era is known as “classical physics,” while the post-Planck era is known as “modern physics.”
Ch. 14: Sugar and Pollen
- Albert Einstein (1879-1955) — A German-born theoretical physicist who is one of the greatest and most influential physicists of all time. Einstein is best known for developing the theory of relativity, but he also made important contributions to thermodynamics and quantum mechanics.
- Einstein and Boltzmann — Einstein’s “miracle year” in which he discovered the theory of relativity was in 1905. But what laid the foundation for that year was his study of Boltzmann’s ideas about entropy. Boltzmann’s statistical explanation of the entropy of a gas influenced Einstein a lot.
- On a Heuristic Point of View Concerning the Production and Transformation of Light — One of Einstein’s 1905 papers in which he uses Boltzmann’s statistical explanation of the entropy of gas to explain how light works. By assuming that a gas consists of tiny lumps and molecules that are in constant motion, Boltzmann had showed that its entropy will increase by chance alone (because molecules remaining in clumps is statistically unlikely). Einstein uses these arguments to show that the way the entropy of light changes can also be explained if it is considered, like a gas, to consist of discrete particles. Just as the air in the room you’re sitting in is made up of tiny particles, so, too, is the light that illuminates it.
- Takeaway — Einstein was basically trying to say that light, like gas, is made up of trillions of tiny particles that are in constant motion. These light particles act like gas particles when it comes to entropy.
- Brownian Motion — A term used to describe the weird behavior of tiny dust like particles in water. In 1905, Einstein used Brownian Motion to confirm Boltzmann’s belief that atoms and molecules are real. Remember, when Boltzmann initially came out with his findings on entropy, many people didn’t believe him because, at that time, nobody believed that atoms and molecules were real. Einstein believed Boltzmann and used Brownian Motion to prove that atoms and molecules were real.
- Drunkard’s Walk — The Drunkard’s Walk is a term applied to Brownian Motion that says that dust/pollen particles in water move randomly, much like a drunk person might stumble along in a town square. Using the Drunkard’s Walk formula, Einstein showed that a pollen particle a thousandth of a millimeter across, drifting in water at 17°C, will move six-thousandths of a millimeter every 10 seconds in some random direction. Einstein was basically saying that if atoms and molecules are real, it’s possible to make a numerical prediction that can be confirmed or if you did by experiment.
- Jean Perrin — In 1909, Jean Perrin, an experimental physicist working in Paris, used Einstein’s prediction to test and confirm Einstein’s theory on Brownian Motion, which therefore confirmed Boltzmann’s original idea that molecules and atoms are real.
- Drunkard’s Walk — The Drunkard’s Walk is a term applied to Brownian Motion that says that dust/pollen particles in water move randomly, much like a drunk person might stumble along in a town square. Using the Drunkard’s Walk formula, Einstein showed that a pollen particle a thousandth of a millimeter across, drifting in water at 17°C, will move six-thousandths of a millimeter every 10 seconds in some random direction. Einstein was basically saying that if atoms and molecules are real, it’s possible to make a numerical prediction that can be confirmed or if you did by experiment.
Ch. 15: Symmetry
- Noether Theorem — States that every differentiable symmetry of the action of a physical system with conservative forces has a corresponding conservation law. The theorem was proven by mathematician Emmy Noether in 1915 and published in 1918.
Ch. 16: Information Is Physical
- Information & Thermodynamics — Energy is needed to drive the machines that process and send our information, and that energy ends up as waste heat, which is dissipated into the planet’s oceans and atmosphere. Every time you search the Internet, the oceans and atmosphere become a tiny bit warmer. The energy required to perform 100 or so Google searches would heat the water needed to make a cup of tea. Essentially, processing information on our devices increases the world’s entropy — making the world a little warmer.
- Ex. Phones — The microchips and hardware that allow our computers and phones to work give off heat when we use them. This is why a phone can feel very hot at times after several hours of intense use, such as watching YouTube videos.
- Quote (P. 184): “In the modern world, the thermodynamic cost of information is determined by the electrical properties of silicon. Typically, every time a single transistor switches between on and off to answer a yes/no question, it dissipates around 10 million-millionths of a joule of heat into its surroundings. That’s a small amount. But consider that in a single chip 10 million transistors are switching on and off a billion times a second. That means that a chip with a surface area of one square centimeter can easily dissipate heat at a rate of several tens of joules per second. If left uncooled, the chip’s surfaces would become hotter than the hot plates on stoves.”
- AT&T — In the early 1900s, AT&T was struggling. The company decided to bring in scientists and physicists to help it meet the challenge of long-distance telephony. By 1915, the company had designed thermionic valves, which traveled along 3,000 miles of copper wire held in place by 130,000 wooden poles that spend the American continent. These developments enabled global communication via cables or wirelessly with the use of radio waves.
- Bell Labs — This success led AT&T’s management team to open Bell Labs in 1925, a giant research and development plant in Manhattan where 2,000 technical experts worked on product development and another 300 worked on basic and applied research. The innovations that came out of Bell Labs helped revolutionize communication.
- Claude Shannon (1916-2001) — Was an American mathematician, electrical engineer, and cryptographer known as a “father of information theory.” Shannon began working in Bell Labs in 1941 and worked on a number of high-stakes projects.
- SIGSALY — One of the projects Shannon worked on was SIGSALY, a top-secret mission where a small team designed a system for encrypting wireless telephone calls in order to communicate without the Germans picking up on the information. The goal was to create a secure encrypted transatlantic communication channel for the Allied Forces in World War II. The first SIGSALY call between London and Washington took place on July 15, 1943. Over the course of the war, SIGSALY enabled about 3,000 high-level telephone conversations, including some between Roosevelt and Churchill.
- Interesting Fact — ‘Enigma’ was the name of the encryption system used by the Germans to protect their military communications in World War II.
- Interesting Fact — There are 200 billion stars in the Milky Way.
Ch. 17: Demons
- Landauer Limit — Named after its discoverer, Rolf Landauer. While at IBM, Landauer studied information and entropy. He found that computer processing requires that information be erased in a microchip, which causes the chip to give off heat and increase the world’s temperature and entropy.
- Quote (P. 196): “The Landauer Limit tells us that however good the technology we create to process bits, once we start erasing those bits, we will make the environment a little warmer. By how much? At temperatures common on the earth’s surface, the amount of heat that will be dissipated when even a perfect storage device erases a bit of information is 3,000 billion-billionths of a joule.”
- Takeaway — The Landauer Limit tells us that we can never create a computer that doesn’t give off some heat when it’s computing or processing data. The best we can do is strive for the 3,000 billion-billionths of a joule number that Landauer gave. The amount of heat our silicon-based technology chips currently give off is much greater than that number, so we have a ways to go before we perfect our chips and the amount of heat/energy they give off.
- Quote (P. 196): “We may never build a useful computer that erases bits at a rate of heat dissipation as low as the Landauer Limit, but knowing it tells us that we can, in principle, reduce the heat coming off our chips by factors of thousands if not millions.”
- Quote (P. 196): “The Landauer Limit tells us that however good the technology we create to process bits, once we start erasing those bits, we will make the environment a little warmer. By how much? At temperatures common on the earth’s surface, the amount of heat that will be dissipated when even a perfect storage device erases a bit of information is 3,000 billion-billionths of a joule.”
Ch. 18: The Mathematics of Life
- Alan Turing (1912-1954) — Was an English mathematician, computer scientist, logician, cryptanalyst, philosopher, and theoretical biologist. He was a historic code breaker, and is most known for helping Britain decode Germany’s encryption system, Enigma, in World War II. He made major contributions to biology and thermodynamics as well.
- The Chemical Basis of Morphogenesis — A 1951 paper written by Turing in which he tried to explain how embryos are shaped as they develop in the womb. Turing was trying to explain how embryos, which start off as a single cell — the fertilized egg known as the zygote — can divide into multiple, identical cells, which later differentiate into the specialist cells that, arranged in highly organized ways, make up a living organism. He was essentially trying to explain how cells, which start out as identical, are able to follow orders and shape themselves so they correctly form the different parts of our body. For example, how do the cells know how to make a hand in the correct spot rather than a foot? That’s what be was attempting to explain. There were a few main ideas in the paper:
- Dissipation — To this point, dissipation was seen as a bad thing that led to death and decay. The second law of thermodynamics discusses entropy and how energy dissipates — such as heat flowing from hot to cold — until the organism is basically dead. Turing was arguing that diffusion could actually help create structure and form.
- Feedback — Turing argued that feedback, both positive and negative, can occur when certain chemicals react, including morphogens in the body. He then argued that when chemicals of this kind diffuse through a body of identical cells, they can trigger changes in them that, as the cells differentiate, causes them to form a recognizable pattern.
- Ex. Zebra Stripes — The skin cells of a zebra start off identical. But as morphogens capable of feedback diffuse through them, they cause some cells to turn dark and others to turn white so that a striped pattern emerges.
Ch. 19: Event Horizon
- Theory of Relativity — Einstein published his ‘special theory of relativity’ in 1905 and his ‘general theory of relativity’ in 1915. Einstein’s theory of relativity overturned Isaac Newton’s theory of gravity, which had been published in 1687. Objects in free fall come down at the same rate of acceleration, even if the size and weight of each object is far different. So, if two different objects are dropped from the roof at the same exact time, they will hit the ground at the same time. That’s gravity.
- Einstein & Newton’s Theory of Gravity — Newton had argued that a gravitational force pulls objects down towards the earth’s center on basically a straight line. Einstein essentially argued that large objects in space (like the earth’s mass) curves space. Earth’s mass has curved space in such a way that a straight line in this curved space leads towards the earth’s center. To test the validity of this, astronomers in 1919 measured star light during an eclipse and found that the light bends around the sun because the space near a massive object like the sun (or earth) is curved. So, basically, the light follows a curved path because the space around the sun is curved. Newton’s law of gravity did not assume that light or objects followed curved paths — this was one of the big findings of Einstein’s theory of relativity.
- Principle of Equivalence — This term was included in Einstein’s papers. An object in complete free fall will encounter the same experience as if the object was in a region of zero gravity. Basically, a person or object in free fall feels no gravity.
- Black Holes — Black Holes are regions of space into which anything can fall but from which (almost) nothing can escape. Black Holes were explained by Einstein’s general Theory of Relativity, which he published in 1915. A good analogy is a powerful drain that sucks nearby water into it. When something gets sucked in, it’s no longer part of our universe.
- Speed of Light — The reason that objects that enter black holes can’t escape is that the black hole is sucking things in at a faster rate than the speed of light. The speed of space flowing around the black hole exceeds the speed of light. Because nothing can move faster than the speed of light, once the object is inside there’s no way to escape.
- Event Horizon — A term given to the ‘one-way door’ of the black hole’s surface that leads objects into the hole and out of our region of space.
- Growth — With each object (i.e. planets, stars, spaceships, etc.) that gets sucked in, a black hole grows in size and mass. As it grows, the black hole’s pull on the flow of space around it increases. Essentially, a black hole can keep getting bigger and bigger and therefore suck in objects from a greater distance. And because nothing can leave the black hole, there’s no way it can get smaller.
Epilogue
- Greenhouse Gases — The discovery, and further study, of thermodynamics has improved our quality of life tremendously. But it has also brought some stress on the planet. In the early 1960s, John Tyndall, an Irish physicist, ran some experiments. His findings led him to the discovery of what we now call ‘greenhouse gases.’
- Infrared Radiation — The sun’s energy reaches us mainly as visible light, which in turn warms the soil and the water on the earth’s surface. The water and soil then radiate some of that energy back into the atmosphere. But the form of the energy that radiates back out of the earth is rather different than visible light. This emerges as infrared radiation. Tyndall wanted to know how infrared radiation behaves in the earth’s atmosphere. Is it trapped or blocked in the earth’s atmosphere? This was an important question because if it couldn’t escape back into space, this infrared radiation would act to warm the atmosphere.
- Findings — Nitrogen and oxygen make up 99% of the atmosphere. Air containing water vapor and carbon dioxide make up only a tiny amount of the atmosphere. But water vapor and carbon dioxide essentially trap some of the sun’s energy by trapping infrared radiation. These two gases — which we call greenhouse gases — effectively provide a warming blanket around our planet by trapping infrared radiation in the earth’s atmosphere. Therefore, when we release more greenhouse gas — water vapor and carbon dioxide —into the environment, the earth’s temperature will rise because there’s more of it available to trap infrared radiation from exiting.
- Quote (P. 243): “Tyndall had discovered what we now call the greenhouse effect. Water vapor and carbon dioxide in our atmosphere trap some of the sun’s energy. Effectively, they provide a warming blanket around our planet. Without these gases in our atmosphere, the earth’s temperature would plummet and would be well below zero even at the equator. But what happens if the levels of these greenhouse gases — the water vapor and the carbon dioxide — go up? Clearly, more heat radiating out from the earth will be trapped, and the earth’s temperature will rise.”